Understanding Oxidative Stress Detection Kits: A Comprehensive Guide
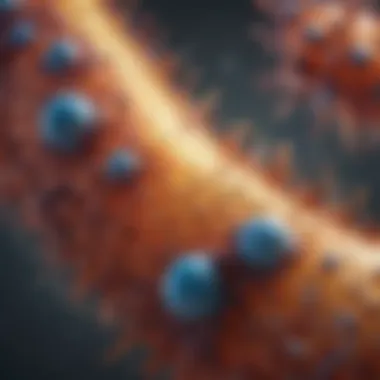
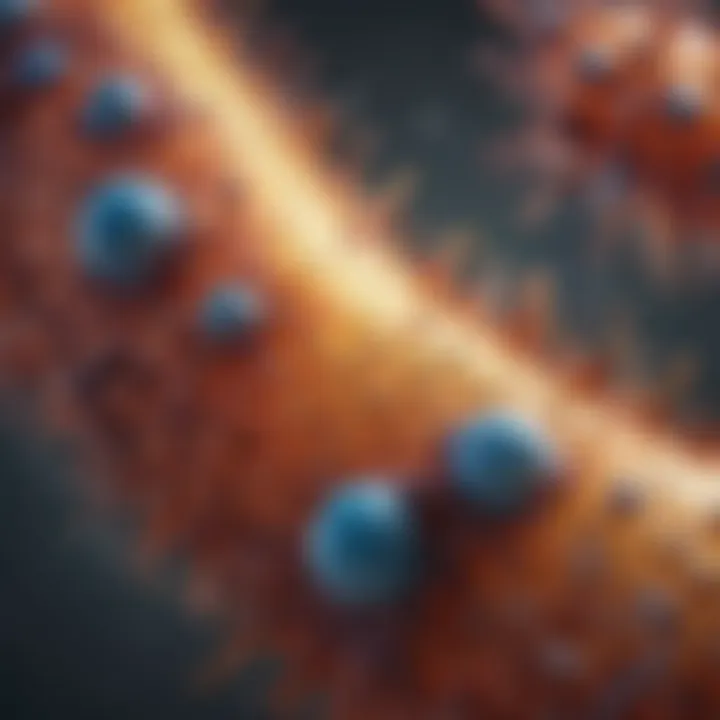
Intro
Oxidative stress is a fundamental aspect of many biological processes and diseases. Understanding its implications is crucial for researchers and healthcare professionals. Detection kits that assess oxidative stress levels are essential tools in this regard. They help in diagnosing diseases, monitoring therapeutic efficacy, and advancing scientific understanding.
In this article, we will explore the methodologies employed in oxidative stress detection, the types of kits available, and their relevance in clinical and research settings. By examining these factors in detail, we aim to provide a comprehensive view of oxidative stress detection kits and their importance in todayβs scientific landscape.
Research Methodology
Understanding the methodologies behind oxidative stress detection kits is vital. This section outlines the research design and approach commonly employed in studies focused on these kits, as well as the materials and methods used.
Description of Research Design and Approach
Research on oxidative stress detection often utilizes a quantitative approach. Studies typically focus on evaluating the performance of various detection kits through controlled experiments. These experiments aim to establish parameters such as specificity, sensitivity, and the range of detectable oxidative stress markers. Researchers perform comparative analyses using different kits to identify the most reliable options for various applications.
Materials and Methods Used in the Study
Materials commonly used in this research include blood samples, tissue samples, and various biochemical reagents. Common oxidative stress markers like malondialdehyde and protein carbonyls are often analyzed. Techniques such as spectrophotometry, fluorometry, and chemiluminescence are utilized to measure the levels of these markers.
The methodologies also depend on the type of detection kit being used:
- Colorimetric Assays: Involve the reaction of oxidized substances to produce a color change that can be measured.
- Fluorescent Assays: Utilize fluorescent markers that react with oxidative stress markers.
- Electrochemical Methods: Measure current changes resulting from redox reactions related to oxidative stress.
Importance of Standardization
Standardization across these methods is critical. It ensures that results are reproducible and comparable across different studies. For this reason, many researchers adhere to guidelines set forth by organizations like the International Society for Redox Biology.
Discussion and Interpretation
Understanding the interpretation of results from oxidative stress detection kits is crucial for their effective application. This section delves into how these results fit into existing literature and what they imply for future research.
Interpretation of Results in the Context of Existing Literature
Results from oxidative stress detection often demonstrate correlations with various diseases such as cancer, cardiovascular diseases, and neurodegenerative disorders. Interpretation of these results must consider confounding factors like age, sex, and underlying medical conditions that can influence oxidative stress levels.
Recent studies indicate a significant relationship between oxidative stress and inflammation, suggesting that oxidative stress may be both a cause and a consequence of various pathological conditions. This duality enhances the need for precise detection methodologies to establish clearer causative relationships.
"Oxidative stress detection is not just a reflection of the current state of health; it can also provide insights into potential future health risks."
Implications for Future Research or Practical Applications
The implications of oxidative stress detection are vast. There is a growing demand for more efficient and user-friendly detection kits. Future developments may focus on integrating technology such as smartphone applications for real-time monitoring of oxidative stress. Moreover, personalized medicine may benefit from understanding an individual's oxidative stress levels, ultimately improving patient outcomes.
As we advance our understanding of oxidative stress, it becomes increasingly important to refine our detection methodologies, ensuring they are as accurate and accessible as possible.
Prolusion to Oxidative Stress
Oxidative stress is a significant area of study in both biomedical research and clinical diagnostics. Understanding this concept is essential for grasping the implications it has on health and disease. In this section, we will delve into the definition of oxidative stress, the mechanisms that lead to its occurrence, and the consequences it can have on physiological systems.
Definition of Oxidative Stress
Oxidative stress refers to an imbalance between the production of reactive oxygen species (ROS) and the antioxidant defenses of the body. Reactive oxygen species are highly reactive molecules that can cause damage to lipids, proteins, and DNA. When the bodyβs antioxidant mechanisms are overwhelmed, this imbalance becomes detrimental. The concept underscores the importance of oxidative stress as a contributing factor to various pathological conditions.
Mechanisms Leading to Oxidative Stress
The mechanisms that lead to oxidative stress are complex and multifaceted. Several factors contribute to increased ROS production, including:
- Environmental factors: Pollution, UV radiation, and toxic substances can enhance ROS formation.
- Metabolic processes: Cellular respiration generates by-products that can lead to oxidative stress if not adequately managed.
- Lifestyle choices: Smoking, excessive alcohol consumption, and poor dietary habits increase oxidative stress.
- Pathological conditions: Infections and chronic inflammation can also boost ROS levels, resulting in cellular damage.
Understanding these mechanisms is crucial for developing strategies to prevent oxidative stress and its associated health risks.
Consequences of Oxidative Stress
The ramifications of oxidative stress are extensive and can lead to a variety of chronic diseases. Some of the primary consequences include:
- Cellular damage: Oxidative stress disrupts cellular integrity, affecting vital processes.
- Aging: Accumulated oxidative damage is linked to the aging process, with implications for longevity and health span.
- Chronic diseases: Conditions such as cardiovascular diseases, diabetes, and neurodegenerative disorders have been associated with oxidative stress. This connection emphasizes the need for effective detection and intervention strategies.
The Significance of Detecting Oxidative Stress
Detecting oxidative stress holds significant importance in both scientific research and clinical practice. This relevance stems from the understanding that oxidative stress is a critical underlying factor in various health conditions. Monitoring oxidative stress levels can provide invaluable insights into the pathophysiology of these conditions, allowing for earlier interventions and more personalized treatments.
Relevance to Human Health
The detection of oxidative stress is crucial for assessing human health. Cells in the body undergo constant metabolic processes, which can produce reactive oxygen species (ROS). When production exceeds the body's antioxidant capacity, oxidative stress ensues. This imbalance is associated with various health issues. By measuring oxidative stress levels, healthcare providers can evaluate a person's risk of developing conditions such as cardiovascular diseases, diabetes, and neurodegenerative disorders. Detection methods can aid in identifying oxidant exposure and the effectiveness of antioxidant therapies.
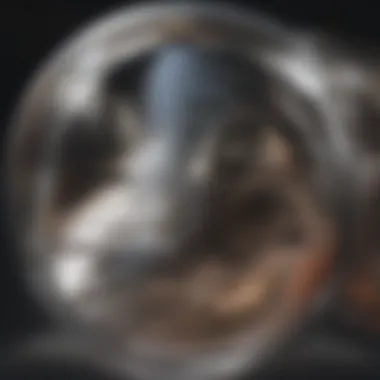
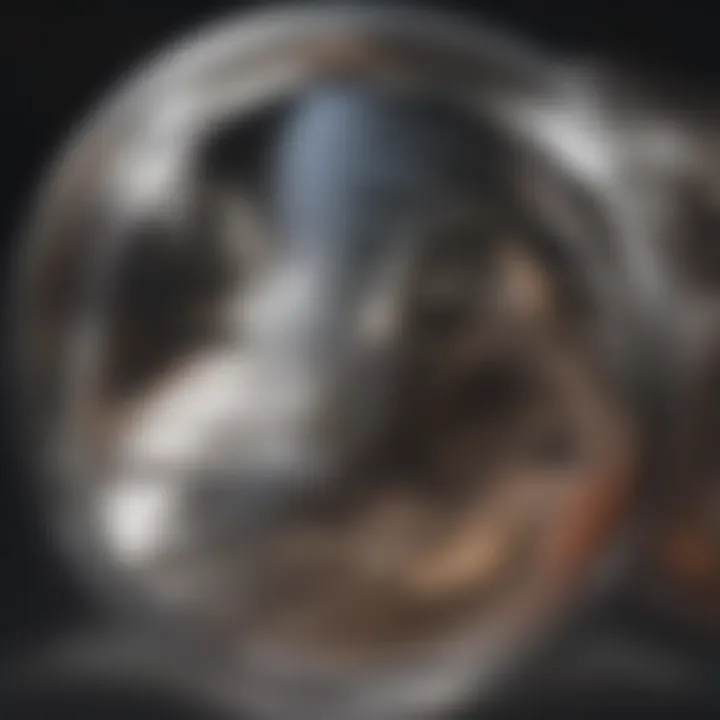
Link to Chronic Diseases
Chronic diseases contribute significantly to global morbidity and mortality, and oxidative stress plays a pivotal role in their progression. Research indicates that many chronic inflammatory diseases, such as rheumatoid arthritis and inflammatory bowel disease, are linked to oxidative damage. The relationship between oxidative stress and these diseases is multifaceted, involving pathway disruptions that affect cellular functions. Early detection of oxidative stress may help in formulating preventive strategies or targeting therapies for managing these conditions, possibly slowing disease progression and improving patient outcomes.
Impact on Aging Process
The impact of oxidative stress on aging is profound. As organisms age, the efficiency of their antioxidant systems often diminishes, leading to an increase in oxidative stress. This unmitigated stress is linked to age-related degeneration of tissues and is thought to contribute to many aspects of aging itself. For instance, oxidative damage to mitochondrial DNA has been implicated in age-related declines in energy metabolism, which can lead to muscle weakness and fatigue. Understanding and detecting oxidative stress in the context of aging can inform strategies aimed at enhancing healthspan, thereby improving quality of life in later years.
Overview of Oxidative Stress Detection Kits
Types of Detection Kits
Oxidative stress detection kits come in several types, each designed for specific applications and methodologies. Common types include:
- Colorimetric kits: Utilize a color change reaction to indicate the presence of oxidative stress markers. They are often simple to use and can be conducted in basic laboratory settings.
- Fluorometric kits: Measure the fluorescence emitted by specific reactions related to oxidative stress. These kits tend to be more sensitive than colorimetric options, offering greater detection capabilities for lower concentrations.
- Electrochemical kits: Use electrochemical methods to quantify oxidative stress markers in samples. They often provide real-time monitoring and high specificity.
Each of these types can offer valuable insights, making them indispensable in various research and clinical contexts.
Components of Detection Kits
The effectiveness of oxidative stress detection kits largely depends on their components. Key components typically include:
- Reagents: Specific chemicals or biomolecules that react with oxidative stress markers in samples. The choice of reagents influences sensitivity and specificity.
- Standards: Reference materials to calibrate the detection process. They provide benchmarks for accurate quantification of oxidative stress levels.
- Instructions and protocols: Clear guidelines facilitate proper usage and ensure reliable results. It is critical that these instructions detail sample preparation, assay steps, and interpretation of results.
Understanding these components aids researchers in selecting the right kits suited for their needs.
Criteria for Choosing a Detection Kit
Selecting an appropriate oxidative stress detection kit requires careful consideration of several factors:
- Sensitivity: Kits should have the ability to detect low levels of oxidative stress markers. High sensitivity improves reliability in detecting subtle changes in samples.
- Specificity: A good kit must accurately distinguish the target markers from other substances present in the sample.
- Ease of use: The simplicity of application is paramount, particularly for non-specialized laboratories. Kits that provide straightforward protocols can yield better outcomes.
- Cost: Budget restraints can dictate which kits are feasible. Researchers should weigh the cost against the kitβs reliability and accuracy.
- Reputation and reviews: Researching the manufacturer and reading user reviews provide insights into the kit's performance in real-world applications.
By adhering to these criteria, researchers and professionals can make informed decisions when selecting oxidative stress detection kits.
Methods of Detection
Understanding the methods of detection for oxidative stress is crucial for both scientific studies and clinical applications. These methodologies allow researchers and healthcare professionals to quantify oxidative stress levels accurately. This insight can lead to better diagnosis and treatment plans for various diseases linked to oxidative stress. Each detection method comes with unique benefits and considerations that make it suitable for different contexts. Choosing the appropriate technique can significantly influence the reliability and validity of the results obtained.
Spectrophotometric Techniques
Spectrophotometry is one of the most common techniques used to measure oxidative stress. It works by measuring the intensity of light absorbed by specific substances in a sample. This technique can offer quantitative data about the levels of reactive oxygen species (ROS) or antioxidants in biological samples.
The reliability of spectrophotometric techniques lies in their ability to provide rapid and reproducible results. They are often straightforward to perform and require relatively uncomplicated equipment. However, it is essential to pay attention to factors like wavelength selection and sample preparation. The accuracy can be affected by the presence of interfering substances. Therefore, careful calibration is necessary for valid outcomes.
Fluorescent Assays
Fluorescent assays have gained popularity due to their high sensitivity and specificity. This method utilizes fluorescent dyes that react with oxidative stress markers, allowing for the detection of low levels of ROS. The intensity of fluorescence emitted corresponds to the concentration of oxidative stress in the sample.
Fluorescent assays offer several advantages, such as real-time monitoring and the ability to perform multi-parameter analyses. However, these methods also pose challenges. Factors like photobleaching can affect the reliability of the results. Moreover, the choice of fluorescent probe must be carefully considered, as different probes respond variably to environmental conditions. Establishing control experiments can help confirm the data collected.
Electrochemical Methods
Electrochemical methods present a novel approach to detecting oxidative stress. These techniques involve measuring the electrical signals generated through the oxidation or reduction of compounds in the sample. Electrochemical sensors often provide real-time data, which is particularly useful in dynamic biological environments.
These methods have the advantage of requiring minimal sample preparation, which can streamline workflows in both clinical and research settings. Along with that, the sensitivity of electrochemical techniques can make them ideal for detecting very low concentrations of oxidative stress markers. Nonetheless, the complexity of electrochemical systems may lead to variability in results, depending on the experimental conditions and the setup used. Ongoing advancements in sensor technology may help overcome these limitations over time.
Factors Affecting Detection Outcomes
In the realm of oxidative stress detection, various factors can significantly influence the outcomes. Understanding these elements is vital for both research and clinical practices. The results from oxidative stress detection kits can vary based on multiple considerations including sample types, environmental conditions, and potential interference from other substances. Recognizing how these factors interplay is essential for reliable data interpretation.
Sample Type and Preparation
The type of biological sample used in oxidative stress detection greatly impacts the results. Different sample types, such as blood, urine, or tissue, can yield varying levels of biomarkers indicative of oxidative stress. For instance, blood samples may reflect systemic oxidative stress, while urine samples can indicate oxidative damage at the cellular level. Each of these samples requires specific preparation techniques to maintain integrity and limit degradation.
It is crucial to consider the following when preparing samples:
- Collection Method: The technique for sample collection can introduce variability. For example, using anticoagulants in blood samples is essential to prevent coagulation, which might affect downstream analysis.
- Storage Conditions: Samples not handled properly might lose viability. Freezing or refrigerating samples can help preserve their state before analysis.
- Processing Time: Delays in processing can lead to significant changes in sample composition. Timely analysis is imperative.
Environmental Conditions
The environment in which oxidative stress detection occurs plays a critical role in the accuracy of the results. Laboratory conditions, including temperature, humidity, and light exposure can affect chemical reactions involved in detection methodologies. Generally, most detection assays require controlled conditions to yield valid outcomes.
Some environmental considerations include:
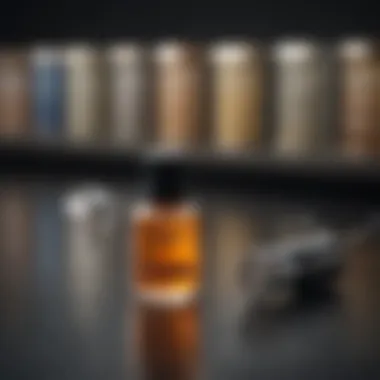
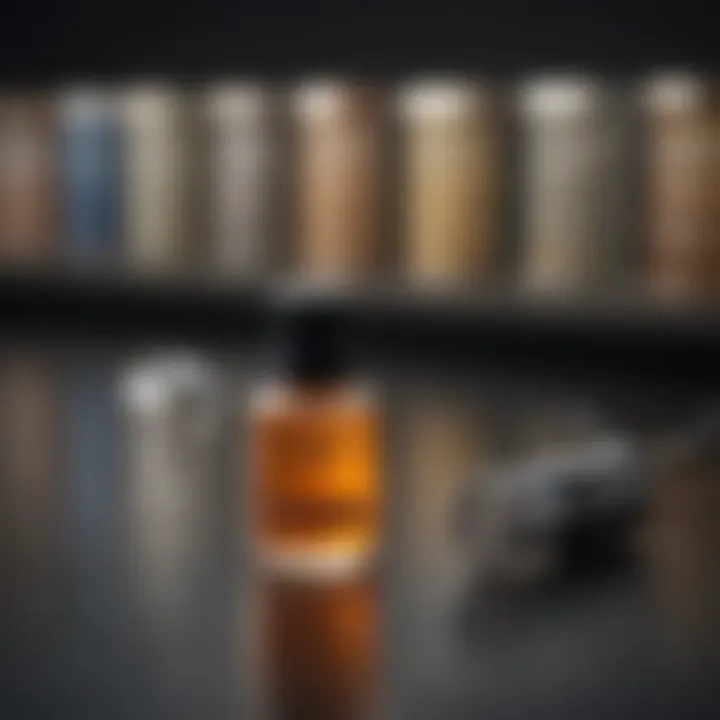
- Temperature: Reactions often have optimal temperatures for activity. Elevated or reduced temperatures can alter the sensitivity and specificity of detection kits.
- Light Exposure: Certain assays are sensitive to light. Prolonged exposure may cause photodegradation of reagents, destabilizing results.
Maintaining stable environmental conditions ensures that the experiments yield reproducible and trustworthy results.
Interference from Other Substances
Interference from external substances is a notable challenge in oxidative stress assays. Many biological samples contain substances that can either stimulate or inhibit the detection reactions. This interference can lead to false positives or negatives, thus skewing the interpretation of oxidative stress levels.
Key substances that may cause interference include:
- Endogenous Biomolecules: Compounds present in samples, such as enzymes or antioxidants, may interact with assay components.
- Contaminants: Cross-reactivity from environmental or matrix contaminants can produce misleading results.
- Co-administered Drugs: Medications affecting redox status can alter oxidative stress measures, necessitating careful consideration of patient medication history.
In summary, recognizing and addressing these factors is imperative for achieving accurate detection outcomes in oxidative stress studies. By optimizing sample types, environmental conditions, and accounting for interferences, researchers can enhance the reliability of their findings.
Comparison of Popular Detection Kits
In the realm of oxidative stress research and clinical diagnostics, several detection kits stand out for their efficacy and reliability. Comparing these kits is crucial for researchers and healthcare professionals who wish to select the most suitable option for their needs. Each kit has unique features, limitations, and specific contexts in which it excels. Understanding these elements contributes significantly to informed decision-making in both laboratory and clinical settings.
Kit A: Features and Limitations
Kit A is designed with high sensitivity and specificity for detecting oxidative stress markers. One significant feature is its ability to provide quantitative results, enabling precise measurement of oxidative stress levels. This kit utilizes a colorimetric technique which simplifies the detection process and makes it adaptable to various laboratory environments. However, one limitation is its relatively high cost when compared to other available options, which may restrict access for smaller laboratories or clinics.
Key Features of Kit A:
- High sensitivity and specificity.
- Quantitative result capabilities.
- Colorimetric detection.
Key Limitations of Kit A:
- Higher cost compared to competitors.
- Requires additional training for optimal use.
Kit B: Features and Limitations
Kit B offers a user-friendly approach with a robust design. It is particularly noted for its rapid detection times, providing results in a shorter window than other kits. This feature makes it appealing for urgent clinical assessments. Additionally, Kit B allows for multiple sample analyses simultaneously, enhancing throughput in busy laboratories. Nonetheless, it may have slightly lower sensitivity in some assays, which could lead to underestimating oxidative stress levels in specific cases.
Key Features of Kit B:
- Rapid detection times.
- Capability for simultaneous sample analysis.
Key Limitations of Kit B:
- Potential lower sensitivity in certain assays.
- Limited range of oxidative stress markers compared to others.
Kit C: Features and Limitations
Kit C distinguishes itself with a comprehensive detection range, covering various markers associated with oxidative stress. This broad scope makes it suitable for diverse research contexts. The kit employs both fluorescent and colorimetric methods, allowing users to choose based on their preferences or laboratory capabilities. However, the dual-mode functionality may complicate its use, as careful calibration and method selection are necessary for optimal results. Furthermore, the intricate protocols may deter less experienced users.
Key Features of Kit C:
- Broad range of oxidative stress markers.
- Options for fluorescent and colorimetric methods.
Key Limitations of Kit C:
- Complex protocols requiring careful adherence.
- May be intimidating for users without extensive training.
It's essential to choose an oxidative stress detection kit that aligns with specific research needs and conditions. Understanding the unique features and limitations can greatly influence the outcomes of your studies or diagnostics.
Applications of Oxidative Stress Detection Kits
Oxidative stress detection kits serve pivotal roles across various domains, particularly in clinical diagnostics, biotechnology research, and environmental assessments. Understanding these applications is crucial as it allows researchers and healthcare professionals to harness the full potential of these kits. Each application provides unique insights and benefits that contribute to health and research advancements.
Clinical Diagnostics
In the realm of clinical diagnostics, detecting oxidative stress is paramount for identifying various health conditions. Oxidative stress is increasingly recognized as a key player in numerous diseases, including cardiovascular conditions, diabetes, and neurodegenerative disorders. By using specific detection kits, healthcare professionals can measure oxidative markers to assess a patient's health status.
The benefits are significant:
- Early Detection: Identifying oxidative stress levels can lead to early diagnosis of health issues, allowing for timely interventions.
- Personalized Treatment: Results can inform tailored treatment plans that address the specific oxidative stressors affecting the patient.
- Monitoring Disease Progression: Regular assessments can help track the progression of diseases over time, indicating the effectiveness of treatment strategies.
Research in Biotechnology
In biotechnology research, oxidative stress detection kits support a wide range of applications, from fundamental research to product development. Researchers use these kits to investigate cellular and molecular responses to oxidative stress, gaining insights into disease mechanisms and therapeutic targets.
Some critical aspects include:
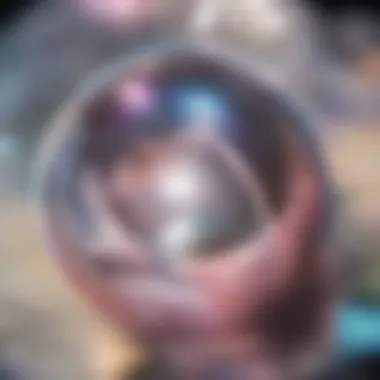
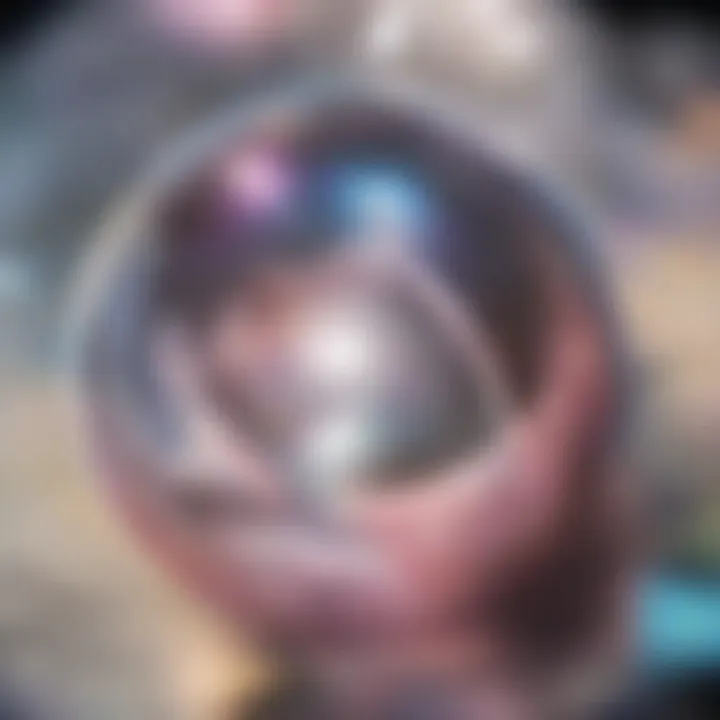
- Understanding Cell Responses: By measuring oxidative stress, scientists can explore how cells respond to environmental stressors and how this response influences health.
- Development of New Therapies: The data collected can guide the formulation of pharmaceuticals that combat oxidative stress-related diseases.
- Evaluating Antioxidant Efficacy: Researchers can assess how effective antioxidants are in reducing oxidative stress and preventing associated damage.
Assessing Environmental Impact
The assessment of oxidative stress extends to environmental studies where detection kits are essential. Environmental stressors, such as pollutants and toxins, can induce oxidative damage in living organisms. By utilizing these detection kits, researchers can evaluate how different substances impact ecological health.
Key considerations in this application include:
- Ecotoxicological Studies: These kits allow for studying the effects of chemicals on wildlife, helping to establish safety guidelines for environmental health.
- Monitoring Pollutants: Assessment of oxidative stress in organisms can provide insights into the effectiveness of policies aimed at reducing pollution and protecting ecosystems.
- Public Health Implications: Understanding how environmental factors contribute to oxidative stress can guide public health initiatives and improve community health strategies.
"Applications of oxidative stress detection are diverse, emphasizing their importance in healthcare, research, and environmental protection."
Challenges in Oxidative Stress Detection
Detecting oxidative stress is vital for understanding many biological processes and their implications for health. However, various challenges hinder the accuracy and reliability of oxidative stress detection kits. Highlighting these issues is essential for researchers and healthcare professionals to navigate the complexities of measurement and interpretation effectively.
Variability in Results
One significant challenge in oxidative stress detection is the variability in results obtained from different assays. Variability can be due to numerous factors including the biological sample type, assay methods, and even the operator performing the tests. For example, variations in the concentration of reactive oxygen species could result in markedly different readings. This variability makes it difficult to compare data across studies or achieve consistent results in clinical settings. Moreover, it can lead to misinterpretation of the presence and extent of oxidative stress, ultimately affecting diagnosis and treatment strategies.
Standardization Issues
Standardization of procedures is another critical concern. Ideally, all detection kits should use standardized protocols to ensure comparability and reproducibility of results. However, many kits have different methodologies and conditions for usage, leading to inconsistencies. Without agreed-upon guidelines, results can differ significantly between labs and studies. This lack of standardization can impede the advancement of knowledge in oxidative stress research, as variations make it challenging to reach consensus on findings or develop universally accepted diagnostic criteria. Variability in detection methods has the potential to complicate regulatory submissions as well, affecting product approval.
Limited Awareness and Knowledge
Lastly, limited awareness and knowledge surrounding oxidative stress detection methods pose a barrier to effective utilization of these kits. Many healthcare professionals and researchers may not fully understand the underlying principles of these detection methods or the potential sources of error. This lack of knowledge can lead to improper use of kits or misinterpretation of results. Education and training are necessary to help overcome these barriers and ensure that oxidative stress detection is utilized to its fullest potential. Further outreach about the available technology and its significance is crucial to enhance the overall quality of research and clinical outcomes.
"Inadequate awareness can directly affect the fidelity of research data and clinical diagnosis."
Overall, addressing these challenges is essential to improve the reliability of oxidative stress detection kits and enhance their application in clinical and research settings. Focusing on standardization, reducing variability, and increasing awareness will drive forward the field's capacity to tackle oxidative stress-related health issues.
Future Directions in Detection Technology
As the field of oxidative stress research advances, the future of detection technology holds significant promise. This section delves into essential innovations and their implications for enhanced detection accuracy and application versatility. With growing recognition of oxidative stress in various health-related phenomena, such developments are crucial for both clinical and research settings.
Emerging Techniques
New methodologies for detecting oxidative stress are constantly being introduced. Advanced techniques, such as nanosensors, offer higher sensitivity and specificity than traditional kits. These sensors operate on the principle of detecting chemical changes at a molecular level. For instance, nanoscale electrodes can measure reactive oxygen species with remarkable precision. The integration of nanotechnology in detection kits can also lead to faster results, a vital factor in clinical diagnostics.
Advantages of emerging techniques include:
- Higher sensitivity and specificity
- Rapid response times
- Potential for real-time monitoring
Integration with Biotechnology
The intersection of biotechnology and oxidative stress detection is becoming increasingly relevant. Techniques like CRISPR and gene editing provide innovative ways to understand oxidative mechanisms in cells. For example, targeted gene editing can be utilized to knock out oxidative stress response pathways, offering insight into their function. Additionally, bioluminescent proteins can be incorporated in detection kits to facilitate visualization of oxidative stress in live organisms. Such integrations not only enrich the study of oxidative stress but also pave the way for tailored therapeutic approaches.
Factors worth considering in integration include:
- Ethical implications of biotechnological applications
- Quality control of biological components
- Regulatory compliance for clinical use
Commercialization Trends
As awareness of oxidative stress grows, so does the market for detection kits. Companies are increasingly seeking to develop user-friendly products that cater to both research and clinical communities. This trend is evident in the rising demand for portable detection solutions. An emphasis on ease of use is paramount, as researchers and healthcare professionals require technology that can deliver reliable results with minimal technical expertise. The collaboration between technology companies and academic institutions can foster innovation, driving the development of kits that are not only effective but also accessible to users.
"The future of oxidative stress detection will likely lean towards more accessible, versatile technologies that can be utilized outside traditional laboratories."
The End
In concluding our exploration of oxidative stress detection kits, it is essential to recognize their fundamental role in both clinical and research contexts. These kits serve as invaluable tools, enabling practitioners and researchers alike to identify and quantify oxidative stress levels effectively. This article has highlighted several crucial elements that underscore their importance:
- Key Implications for Health: Understanding oxidative stress is critical for diagnosing various diseases, crafting treatment plans, and assessing patient health over time.
- Integration in Scientific Research: Researchers increasingly rely on these kits to draw connections between oxidative stress and chronic illnesses, advancing our knowledge and therapeutic methodologies.
- Addressing Challenges: It is evident that the challenges surrounding variability and standardization in detection outcomes demand greater attention. Overcoming these issues can enhance the reliability of results, further validating the utility of these kits.
The implications of this topic extend well beyond simple measurement; they encompass the continual development of more sophisticated methods and technologies that can improve accuracy and expand the applications of these detection kits.
Summary of Key Points
The examination of oxidative stress detection kits reveals several key points:
- Essential for Diagnosis: Accurate detection of oxidative stress is pivotal for understanding disease mechanisms.
- Variety of Kits: Different detection methodologies offer unique advantages depending on the context of use.
- Challenges in Standardization: Variability in results calls for improved standardization practices.
- Future Trends: Emerging detection technologies promise to advance the field significantly.
- Impact on Research: Continued investment in research will yield new insights into the role of oxidative stress in health and disease.
Implications for Future Research
Future research in oxidative stress detection kits should focus on the following areas:
- Development of New Technologies: There is a need for innovative detection methods that offer greater accuracy and efficiency.
- Longitudinal Studies: Conducting comprehensive studies can help clarify the relationship between oxidative stress and chronic diseases.
- Standardization Protocols: Establishing standard protocols will facilitate comparison across different studies, ensuring consistency and reliability.
- Education and Awareness: Increasing knowledge about oxidative stress among healthcare providers will foster better diagnostic practices and treatment outcomes.
"Investing in oxidative stress research today will lay the groundwork for more precise health interventions tomorrow."