Understanding Photovoltaic Cells: Mechanisms and Applications
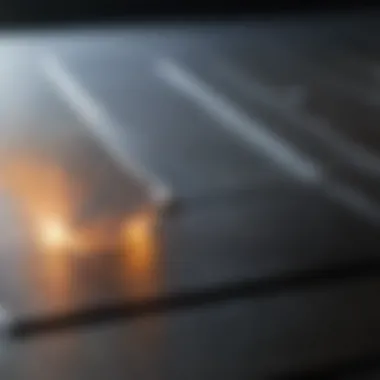
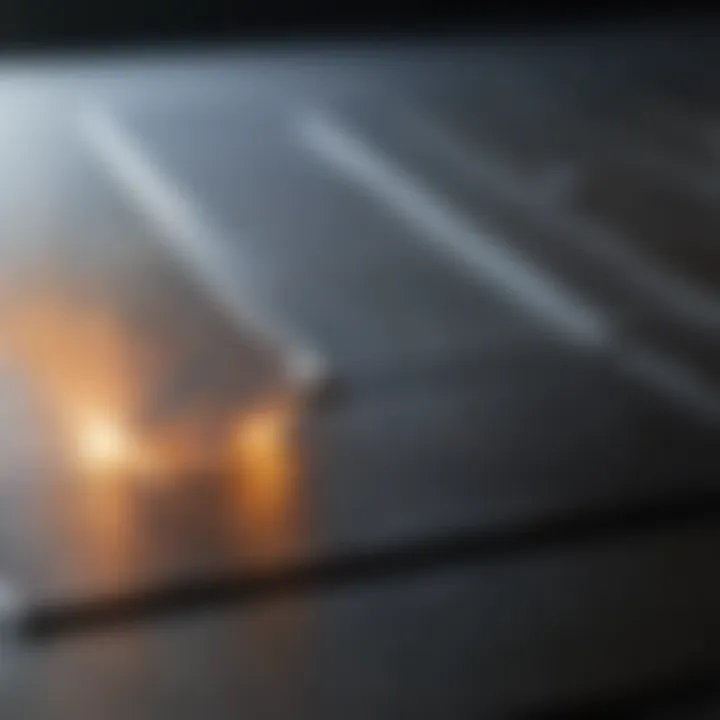
Intro
Photovoltaic cells, commonly known as solar cells, have become an essential component of modern renewable energy technology. As the world grapples with the pressing challenges of climate change and diminishing fossil fuel reserves, understanding these cells becomes increasingly relevant. This section aims to provide a broad overview of photovoltaic cells, their operational mechanisms, and applications in various sectors. The focus on their functionality and efficiency underscores their significance in the move towards sustainable energy solutions.
Photovoltaic cells work by converting sunlight directly into electricity through the photoelectric effect. This process is founded on the fundamental principles of physics and materials science, making it a rich field of study for researchers and students alike. The following sections will delve into specific aspects such as the types of photovoltaic cells available, the factors influencing their conversion efficiency, and the technological advancements that continue to reshape this industry.
In recognizing the environmental impact and economic implications of photovoltaic technology, we can better appreciate its role in addressing global energy demands. This exploration will ultimately provide insights into future prospects within this dynamic field.
Preamble to Photovoltaic Cells
Photovoltaic cells are at the forefront of renewable energy technology. They play a crucial role in transforming sunlight into usable electricity. As the world grapples with the impacts of climate change and dwindling non-renewable resources, understanding photovoltaic technology becomes increasingly important. This section will explore the definition, history, and significance of photovoltaic cells, setting the stage for a deeper examination of their mechanisms and applications.
Definition of Photovoltaic Cells
Photovoltaic cells, commonly known as solar cells, are devices that convert light energy directly into electrical energy through the photovoltaic effect. This effect involves generating electron-hole pairs when photons from sunlight strike the semiconductor material within the cells. When these generated charges are separated by an internal electric field, they create a flow of electric current, which can be harnessed for power. Typically, crystalline silicon is used in the construction of these cells due to its effective conversion properties. Its well-documented efficiency underscores why photovoltaic technology is a preferred choice for clean energy generation.
History of Photovoltaic Technology
The journey of photovoltaic technology began in the 19th century with the discovery of the photovoltaic effect by French physicist Alexandre-Edmond Becquerel in 1839. However, it wasn't until the mid-20th century that significant advancements occurred. In 1954, scientists at Bell Labs developed the first practical silicon solar cell, achieving about 6% efficiency. This milestone led to a surge in interest and investment in solar technology.
As the years progressed, efficiency improved thanks to research and investments in materials science and engineering. The 1970s oil crisis further motivated governments and industries to explore renewable energy sources, including solar power. Today, photovoltaic cells boast efficiencies ranging from 15% to over 22% in commercial products, supported by ongoing research into new materials and methods. As nations strive for sustainable energy solutions, the history of photovoltaic technology provides crucial context for its present and future applications.
"The evolution of photovoltaic cells mirrors humanity's quest for sustainable energy solutions, reflecting both technological advancements and environmental awareness."
Understanding photovoltaic cells is essential not only for academic pursuits but also for practical applications in today's energy landscape. This section lays a foundation for appreciating the complexities and innovations that shape this critical field.
The Science Behind Photovoltaics
Understanding the science behind photovoltaics is crucial for grasping how these systems harness solar energy. Photovoltaic cells convert sunlight into electricity through various mechanisms. Appreciating these mechanisms helps to determine the factors affecting efficiency and contributes to advancements in solar technology.
Fundamentals of the Photoelectric Effect
The photoelectric effect is the phenomenon where light energy can dislodge electrons from a material, usually a metal. This principle underpins the operation of photovoltaic cells. When photons strike the surface of a semiconductor, energy transfer occurs, causing electrons to become excited and ejected from the material.
This process can be summarized in a few critical steps:
- Photon Absorption: The semiconductor absorbs a photon.
- Electron Excitation: The energy from the photon excites an electron to a higher energy level.
- Electron Ejection: The energized electron is released, creating a free electron.
- Electric Current Generation: These free electrons flow through the material, generating an electric current.
The efficiency of this process depends on factors such as the wavelength of the light and the properties of the semiconductor.
Semiconductors and Their Role
Semiconductors are materials that have electrical properties between those of conductors and insulators. Silicon is the most common semiconductor used in photovoltaic cells. Its ability to create a junction between p-type and n-type materials enables effective light absorption and electron movement.
In photovoltaic cells, the doping process is essential. This involves adding impurities to pure silicon to create a positive (p-type) or negative (n-type) area, facilitating charge separation.
Key characteristics of semiconductors include:
- Band Gap Energy: Determines how much energy is needed to free an electron.
- Absorption Coefficient: Measures how well the material absorbs light.
- Carrier Mobility: Refers to how easily electrons move through the material.
Understanding these properties helps improve cell design and efficiency.
How Light Energy is Converted to Electricity
The conversion of light energy to electricity in photovoltaic cells involves more than just the photoelectric effect. It includes charge carrier generation, separation, and collection processes:
- Generation of Charge Carriers: Light energy generates electron-hole pairs.
- Separation of Carriers: Electric fields within the cell push electrons and holes in opposite directions.
- Collection of Charge Carriers: Electrons are gathered at the electrical contacts, leading to the creation of a current.
Efficiency plays a crucial role in this process. Factors such as temperature, light intensity, and angle of incidence can impact the effectiveness of light conversion. Optimizing these elements leads to more efficient photovoltaic technologies.
In summary, a strong understanding of the science behind photovoltaics is essential for advancements in solar energy technology.
Through grasping the photoelectric effect, the role of semiconductors, and the steps for converting light to electricity, one can appreciate the underlying principles that drive solar innovation.
Types of Photovoltaic Cells
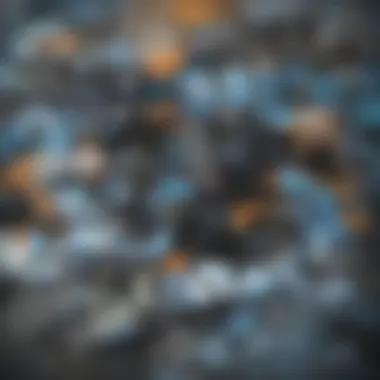
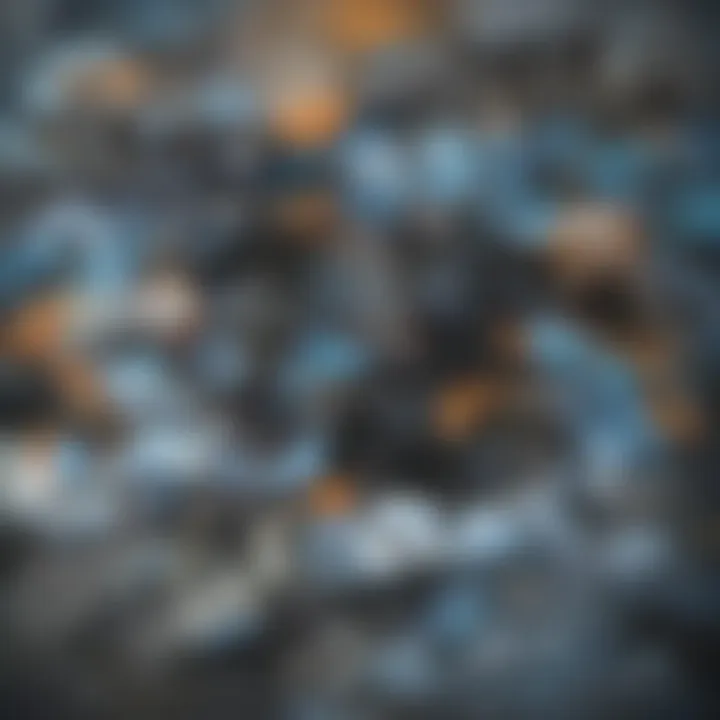
Understanding the types of photovoltaic cells is essential in comprehending how solar energy technology is evolving. Each type of cell has unique features, advantages, and limitations that affect performance, cost, and applicability in various scenarios. As the demand for renewable energy increases, the choice of photovoltaic technology becomes even more critical. Here, we will explore four main types of photovoltaic cells: monocrystalline, polycrystalline, thin-film, and emerging technologies.
Monocrystalline Photovoltaic Cells
Monocrystalline photovoltaic cells are recognized for their high efficiency and sleek appearance. Made from a single crystal structure, typically silicon, these cells offer the highest performance rates among all types. Their efficiency can reach beyond 20%, depending on the technology and manufacturing process used. The advantages of monocrystalline cells include:
- Space Efficiency: Due to their higher efficiency per square meter, monocrystalline cells are ideal for areas with limited space.
- Longevity: These cells often have longer lifespans, commonly exceeding 25 years, which contributes to their cost-effectiveness over time.
- Aesthetic Appeal: Their uniform dark color and sleek design make them more visually appealing for residential installations.
Despite these benefits, there are some considerations. Monocrystalline cells typically come at a higher initial cost. Additionally, they may perform less optimally in high temperatures, which can slightly reduce their overall efficiency.
Polycrystalline Photovoltaic Cells
Polycrystalline photovoltaic cells are a popular alternative to their monocrystalline counterparts. Made from multiple silicon crystals melted together, they have a more complex manufacturing process. Their efficiency generally ranges between 15% to 20%.
The key benefits of polycrystalline cells include:
- Lower Cost: They are usually cheaper to produce, making them accessible for a broader market.
- Sustainable Manufacturing: The process involves less energy than monocrystalline cell production, offering a somewhat lower environmental impact.
- Good Performance in Various Conditions: Polycrystalline cells tend to perform adequately even in lower light conditions.
Nonetheless, there are drawbacks to consider. Polycrystalline cells tend to be less efficient and occupy more space than monocrystalline cells. They may not offer the same aesthetic appeal due to their speckled appearance.
Thin-Film Photovoltaic Cells
Thin-film photovoltaic cells represent a distinct category within solar technology. They are made by depositing a thin layer of photovoltaic material onto a substrate, which can be glass, plastic, or metal. Their efficiency falls between 10% to 12%, generally lower than crystalline technologies.
Advantages of thin-film cells include:
- Flexibility and Weight: These cells are lightweight and can be integrated into various surfaces, including curved structures.
- Performance in High Temperatures: Thin-film cells tend to perform better than crystalline cells in hot conditions.
- Lower Costs: The manufacturing process is often cheaper, which can translate to lower purchase prices for consumers.
However, thin-film cells have their challenges. Their efficiency is significantly lower, requiring more space to generate the same amount of energy as crystalline cells. Also, their lifespan is generally shorter, which may lead to a less favorable cost-benefit ratio over time.
Emerging Technologies in Photovoltaic Cells
The field of photovoltaic technology is evolving rapidly, introducing innovative types of cells that promise improved efficiency and sustainability. Emerging technologies, such as perovskite solar cells, are at the forefront of this revolution. These cells can achieve efficiencies exceeding 25% in laboratory settings.
Features of emerging technologies are:
- High Efficiency Potential: With ongoing research, emerging photovoltaic cells are likely to increase efficiency levels significantly.
- Cost-Effectiveness: Many new materials can be produced at lower costs, making solar energy more accessible.
- Hybrid Technologies: Some emerging technologies combine multiple materials to optimize performance across varying conditions.
While promising, these technologies often remain in the experimental or early deployment stages. Challenges such as stability, environmental degradation, and scalability need further exploration before widespread adoption can occur.
Factors Affecting Efficiency
The efficiency of photovoltaic cells plays a crucial role in determining the viability of solar energy as a sustainable power source. Understanding these factors is essential as they impact the overall performance of solar installations. There are several key elements to consider when assessing efficiency:
- Temperature: It affects the functionality of the cells.
- Light intensity and angle: Directly correlated to energy production.
- Materials and construction: Influences longevity and efficacy.
These factors collectively determine how effectively photovoltaic systems convert sunlight into usable electricity.
Temperature Impact on Performance
Temperature has a significant impact on the performance of photovoltaic cells. As temperature increases, most photovoltaic materials experience a decrease in efficiency. This is primarily due to the inherent properties of semiconductor materials used in solar cells. For instance, monocrystalline and polycrystalline cells generally exhibit a reduction in voltage output as the operating temperature rises.
In warmer climates, this can lead to a notable drop in energy production. Thus, the thermal design of solar installations must be taken into account. Cooling techniques or heat-resistant materials can mitigate these effects and help maintain optimum performance year-round.
Light Intensity and Angle
The amount of sunlight reaching a photovoltaic cell significantly influences its energy output. Light intensity, or irradiance, is directly related to the efficiency of the cells in converting solar energy. Higher light intensity corresponds to greater energy generation. However, it is not merely the quantity of light that matters; the angle at which sunlight strikes the panels also plays a crucial role.
When sunlight hits the surface of a solar cell at a perpendicular angle, the maximum energy absorption occurs. Conversely, when sunlight hits at a low angle, the effective area for absorption diminishes, causing reduced energy conversion rates. Therefore, the installation angle of solar panels is an important factor to optimize.
Materials and Construction
The choice of materials and the construction quality of photovoltaic cells are pivotal for maximizing efficiency. High-quality semiconductor materials, such as silicon, not only improve energy conversion rates but also enhance the durability and lifespan of the solar panels. Techniques such as passivation and anti-reflective coatings can improve the performance by reducing electron-hole recombination and minimizing light reflection.
Moreover, the encapsulation and assembly methods employed can affect how well the cells can withstand environmental aggressors like UV radiation and moisture. As technology continues to evolve, innovations in materials science can lead to even more efficient photovoltaic cells, ensuring advancements in solar power technology remain constant.
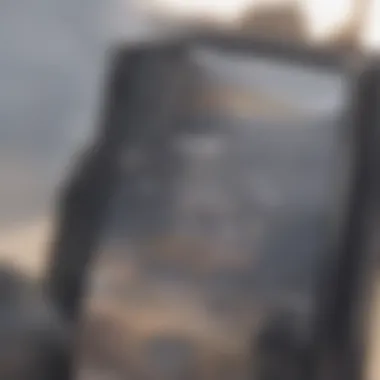
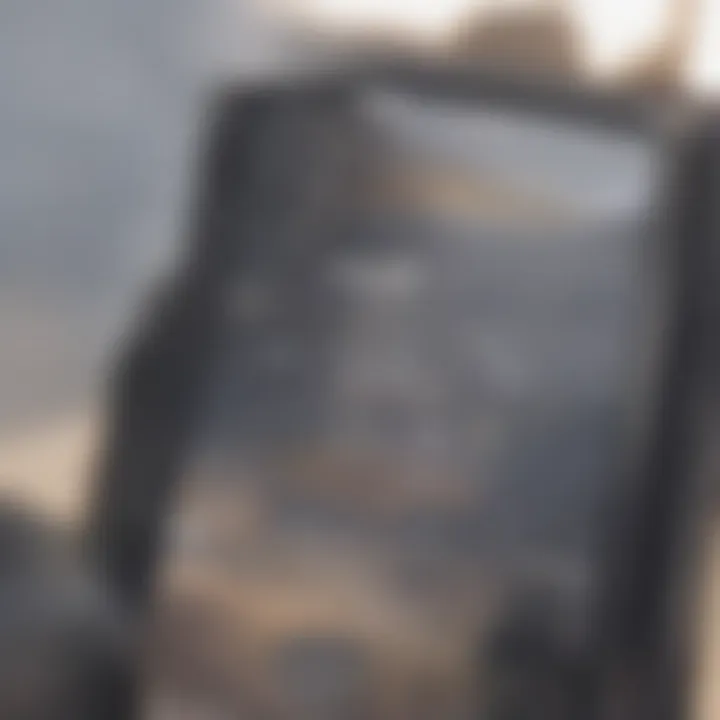
Important Note: For anyone working with photovoltaic technology, attention to these factors can lead to substantial increases in efficiency and, consequently, energy output.
Installation and Applications
The process of installing photovoltaic cells holds substantial significance within the broader context of developing sustainable energy solutions. Effective installation can maximize the efficiency and utility of solar technology. Not only does it promote energy independence, but it also contributes to minimizing greenhouse gas emissions. The applications of photovoltaic cells extend across various sectors, reflecting their adaptability and importance. Understanding these facets can aid stakeholders, including policymakers, businesses, and homeowners, in harnessing solar energy more effectively.
Solar Farms and Utility-Scale Installations
Solar farms represent large-scale installations of photovoltaic systems often found in rural areas. These facilities convert sunlight into electricity on a significant scale, contributing to the energy grid. The capacity of these installations can reach several megawatts, providing a substantial portion of clean energy.
- Benefits:
- Considerations:
- Economies of Scale: Solar farms can produce electricity at a lower cost per unit compared to smaller systems.
- ** land Efficiency**: Large tracts of land can be utilized, optimizing solar collection while reducing land use competition.
- Grid Stability: They enhance grid stability by supplying consistent, renewable energy.
- Location: The placement of solar farms is critical to maximize sunlight exposure.
- Regulatory Framework: Understanding local regulations is crucial for successful implementation.
Residential Solar Panel Systems
Residential solar panel systems provide a tailored solution for homeowners looking to reduce energy costs and their carbon footprint. By converting sunlight directly into electricity, homeowners can generate their own power, leading to a reduction in reliance on fossil fuels.
- Benefits:
- Considerations:
- Cost Savings: Users can significantly reduce their electricity bills.
- Increased Property Value: Homes with solar installations often see an increase in market value.
- Incentives: Many governments provide tax incentives and rebates for residential solar systems.
- Initial Investment: Upfront costs can be substantial, though financing options are available.
- System Size: The size and orientation of the roof will influence the number of panels needed for maximum efficiency.
Integration with Other Energy Systems
Integrating photovoltaic cells with other energy systems can enhance overall efficiency. This approach can lead to improved energy management and resilience in energy supply.
- Hybrid Systems: These systems can combine solar with other renewable sources, such as wind or hydroelectric power, creating a diversified energy portfolio.
- Energy Storage Solutions: Battery storage systems allow excess energy generated during peak sunlight hours to be stored for later use, helping to mitigate intermittency issues.
- Smart Grids: Connecting solar installations to smart grid technology allows for real-time monitoring and management of energy resources, enhancing grid responsiveness and reliability.
"The integration of photovoltaic cells with other energy systems represents not just a technological advancement, but a pathway toward more resilient and sustainable energy infrastructures."
These applications illustrate the versatility and potential of photovoltaic cells in real-world scenarios, highlighting their importance in achieving a sustainable energy future. The dynamics of installation, be it on a utility scale or residential scale, will play a vital role in how effectively solar energy can be harnessed and utilized.
Environmental and Economic Considerations
The significance of environmental and economic considerations in the context of photovoltaic cells cannot be understated. As the world increasingly moves towards sustainable energy solutions, the impact of solar technology on the environment and its economic viability must be assessed carefully. Photovoltaic cells are a crucial component in this transition, and understanding their environmental effects and economic implications is essential for policy makers, industries, and consumers alike.
Lifespan and Recycling of Photovoltaic Cells
The lifespan of photovoltaic cells generally ranges from twenty-five to thirty years. This durability means that after installation, these systems can provide long-term energy solutions with minimal maintenance. However, at the end of their operational life, it is essential to discuss recycling options. The recycling of photovoltaic cells can mitigate waste and recover valuable materials. Some components, such as silicon and silver, can be reclaimed and reused, which contributes to a circular economy.
Additionally, proper recycling processes can reduce environmental hazards. Without recycling, these cells could potentially leach toxic substances into soil and water. Establishing effective recycling programs is crucial to enhancing sustainability further.
Cost-Benefit Analysis of Solar Energy
Conducting a cost-benefit analysis of solar energy involves looking at both the direct and indirect benefits of photovoltaic technology.
- Initial Investment: The upfront cost of purchasing and installing solar panels can be substantial. However, this investment is often offset by long-term savings on electricity bills and, in many cases, government incentives.
- Long-term Savings: Once installed, photovoltaic systems can significantly reduce energy costs. Over time, these savings can surpass initial costs, making solar energy an economically attractive option.
- Social Benefits: Transitioning to solar energy contributes to reduced greenhouse gas emissions, which helps combat climate change. This has far-reaching benefits for public health and environmental quality, leading to lower health care costs and improved quality of life.
Ultimately, moving towards solar energy not only represents a cleaner energy source but also a viable economic strategy.
Regulatory and Policy Framework
The regulatory and policy framework surrounding photovoltaic technology is crucial for its growth and acceptance in the energy market. Many governments have established policies to encourage renewable energy adoption, including:
- Incentives and Subsidies: These can take many forms, such as tax credits or rebates for installations, which significantly lower the barrier to entry for consumers and businesses.
- Renewable Energy Standards: Governments may mandate that a certain percentage of energy comes from renewable sources, bolstering the market for photovoltaic technologies.
- Research Funding: Public investments in solar technology research can lead to significant advancements in efficiency and cost-reduction, making solar energy more accessible.
Understanding the interplay between regulations and market forces will help stakeholders make informed decisions and optimize the growth of photovoltaic technologies.
Challenges Facing Photovoltaic Technology
The photovoltaic industry has transformed significantly in recent decades, but it is not without challenges. Addressing these challenges is key to optimizing the deployment and effectiveness of solar technologies. The pressing issues include intermittency of solar energy, land use implications, and the competitive landscape that includes ongoing market pressures.
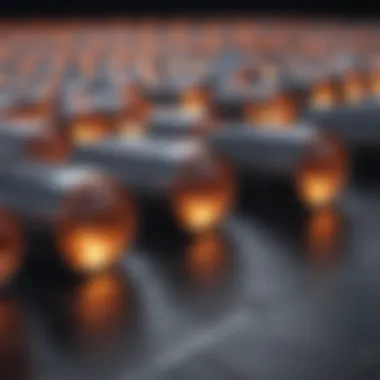
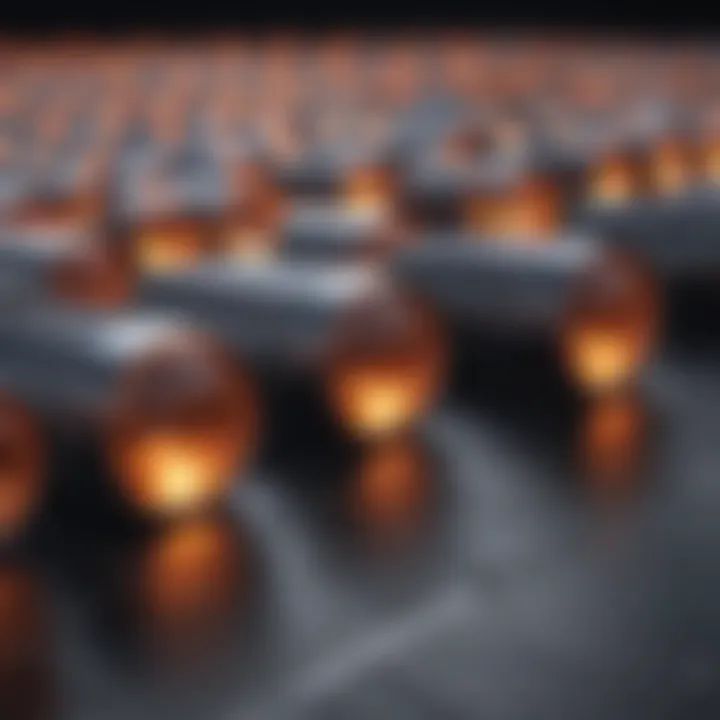
These obstacles carry significant weight in shaping the future of photovoltaic technology. Tackling these challenges could determine the feasibility and sustainability of solar energy as a dominant player in renewable energy. Thus, understanding these elements is essential for students, researchers, educators, and industry professionals alike.
Intermittency and Energy Storage Solutions
One primary challenge in photovoltaic technology is intermittency. Solar energy generation fluctuates due to various factors like time of day and weather conditions. Unlike conventional power plants that can provide consistent energy, solar energy is often not available during night hours or cloudy days. This inherent variability presents a problem for energy grid stability.
To address these efficiency and reliability concerns, energy storage systems are vital. Here are some prevalent solutions:
- Batteries: Lithium-ion and other battery technologies can store excess energy produced during peak sunlight, compensating for periods of low generation.
- Pumped Hydro Storage: This method involves using excess energy to pump water uphill, which can later be released to generate electricity when required.
- Flywheels: These devices store energy in the form of kinetic energy, providing quick releases of energy to balance supply and demand.
"Energy storage technologies are essential to overcoming the intermittency of solar power and maximizing the utilization of photovoltaic systems."
Land Use and Environmental Impact
Land use is another significant challenge in deploying photovoltaic systems widely. Large solar farms require substantial land, sometimes impacting agriculture, wildlife habitats, and local ecosystems. The potential environment impact of these installations requires careful planning and regulation.
Several considerations emerge in this context:
- Impact on Biodiversity: Solar farms can disrupt local habitats. Strategies to mitigate this involve integrating renewable installations with existing ecosystems or using brownfield sites.
- Agrivoltaics: A promising solution is dual land use, where solar panels coexist with agricultural practices. This not only preserves land but also enhances food security.
Additionally, the environmental impact of manufacturing photovoltaic cells requires scrutiny. The lifecycle analysis of materials used in cell production needs to consider recycling and waste management strategies to minimize ecological damage.
Market Competition and Innovation Pressure
The photovoltaic sector is highly competitive. Numerous companies converge on this market, driving improvements in technology and efficiency. However, this creates pressure for innovation and may lead to challenges like price wars. As technology advances, companies must continually adapt, potentially leaving behind those that do not keep pace.
Key factors in this competitive landscape include:
- Cost Reduction: Continuous innovation in production methods is essential to lower costs, making solar energy affordable.
- Patent Wars: Intellectual property in technology advancements can lead to legal battles, hindering progress instead of fostering collaboration.
- Consumer Awareness: As the market grows, public education on the benefits of solar technology becomes vital. This knowledge can drive demand, anchoring photovoltaic products in mainstream adoption.
The Future of Photovoltaic Cells
The future of photovoltaic cells is a critical aspect in the discussion about renewable energy. With the growing concerns about climate change and the demand for sustainable energy sources, photovoltaic technology will play a pivotal role. Its ability to convert sunlight into electricity can significantly reduce dependency on fossil fuels. This section will explore various elements, benefits, and considerations regarding this technology’s future, offering insights into what to expect in the coming years.
Technological Innovations on the Horizon
The advancements in photovoltaic technology continue at a rapid pace. Innovations are emerging, promising to enhance efficiency and reduce costs. Some notable developments include:
- Bifacial Solar Panels: These panels capture sunlight on both sides, increasing energy production. They are being increasingly installed in solar farms.
- Perovskite Solar Cells: Known for their excellent light absorption and easier production, these cells have the potential to revolutionize the industry. Their efficiency rates are already approaching those of traditional silicon-based cells.
- Integrated Photovoltaics: This technology integrates solar cells into building materials, like windows and roof tiles. This provides a dual function, making energy generation easier and less intrusive.
Through these innovations, the efficiency of photovoltaic cells is likely to increase significantly, which will make solar energy more accessible and cost-effective.
Potential for Integration with Smart Grids
The integration of photovoltaic cells with smart grids offers numerous benefits. Smart grids are electricity supply networks that use digital communications technology to detect and react to local changes in usage. This enhances the efficiency and reliability of electricity distribution. Key advantages include:
- Real-Time Monitoring: Smart grids can monitor the generation from photovoltaic cells in real-time, allowing for better energy management.
- Energy Storage Solutions: Coupled with battery storage technology, this integration can solve intermittency challenges by storing excess energy generated during peak sunlight hours for use during cloudy days or nighttime.
- Demand Response Capabilities: The system can adjust energy consumption based on availability, optimizing usage and minimizing waste.
As cities and communities move toward smarter energy solutions, the role of photovoltaic cells in this ecosystem is essential.
Global Trends and Forecasts
Looking globally, the photovoltaic market is expected to grow. Several factors influence this trend:
- Policy Support: Governments are increasingly supportive of renewable energy policies. Incentives for solar energy adoption lead to higher installation rates of photovoltaic systems.
- Economic Factors: As prices for solar technology decline, more consumers and businesses are willing to invest. This trend is expected to continue as production techniques improve.
- Environmental Awareness: Public awareness of climate issues drives demand for clean energy solutions.
Forecasts suggest that the capacity for photovoltaic energy generation will continue to rise. The International Energy Agency estimates that solar photovoltaic capacity could triple by 2030. This growth reflects increased recognition of solar energy’s potential to make a substantial impact on global energy systems.
"The transition to solar energy is not just an option; it is a necessity for a sustainable future."
End
The conclusion of this article encapsulates the extensive exploration of photovoltaic cells, emphasizing their critical role in advancing renewable energy technologies. Overall, understanding the mechanisms, applications, and potential of these cells is vital amidst the current quest for sustainable energy solutions. With the rising demand for clean energy, photovoltaic technology stands out not just for its environmental benefits but also for its long-term economic potential.
Summary of Key Points
- Photovoltaic Cells: These unique devices convert solar energy into electricity through the photoelectric effect.
- Types of Photovoltaic Cells: Monocrystalline, polycrystalline, thin-film, and emerging technologies each have distinct characteristics and applications.
- Efficiency Factors: As noted, temperature, light intensity, and material composition can significantly influence the performance and efficiency of photovoltaic cells.
- Applications: They serve multiple sectors through solar farms, residential systems, and coordination with other energy systems.
- Challenges and Future Directions: Technologies continue to evolve, addressing issues such as intermittency, land use, and market competition. Innovations like smart grids offer promising pathways.
Final Thoughts on Photovoltaic Technology
"Photovoltaics offer a pathway to reduce dependency on fossil fuels, creating a cleaner environment and empowering local energy independence."
This article aims to inform and stimulate further inquiry into photovoltaic technologies, fostering understanding that can drive innovation and application.