Understanding Plasmids: Structure and Applications
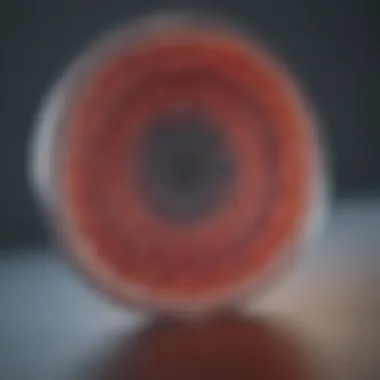
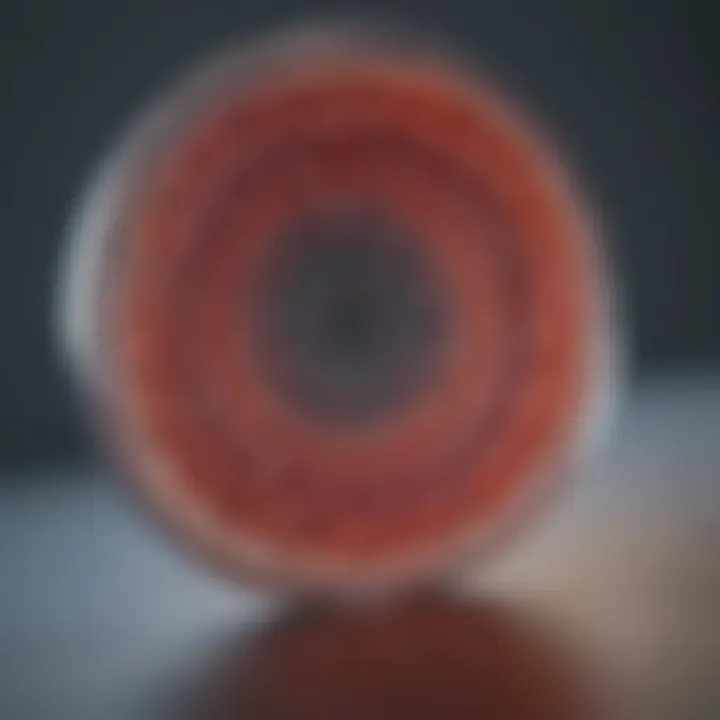
Intro
In the deep waters of microbiology, plasmids often drift beneath the surface, quietly crafting stories that intertwine with the very fabric of genetic engineering and biotechnology. These small, circular DNA molecules hold a treasure trove of information, influencing both microbial life and the scientific approaches that harness their capabilities. As we peel back the layers of plasmids, it becomes essential to grasp not just their structure but also their diverse functions and broad applications that extend from the laboratory into the realms of medicine and agriculture.
Research Methodology
Understanding plasmids isn't merely an academic exercise; it demands a robust approach to research that combines both theoretical study and practical insights.
Description of Research Design and Approach
The journey begins with a thorough review of published literature, which sheds light on the intricate mechanisms by which plasmids operate. By employing a qualitative research design, scholars and scientists alike delve into the historical perspective of plasmid discovery and their evolving functions. This historical lens allows researchers to situate plasmids within their broader biological context, understanding how these genetic elements participated in the grand tapestry of evolution and adaptation.
Materials and Methods Used in the Study
To explore the structural attributes of plasmids, laboratory techniques such as plasmid extraction, restriction enzyme analysis, and gel electrophoresis are frequently employed. These methods facilitate a practical exploration of plasmid diversity and dynamics.
- Plasmid Extraction: Isolate plasmids from bacterial cells to analyze their DNA sequences.
- Restriction Enzyme Analysis: Utilizes specific enzymes to cut DNA at precise locations, aiding in mapping plasmid structures.
- Gel Electrophoresis: Separates DNA fragments, allowing for visualization of size differences and structural alterations.
This interplay of literature review and laboratory experiments enriches our understanding, making it possible to appreciate the multi-faceted nature of plasmids, their mechanisms, and their contributions to various fields.
Discussion and Interpretation
Engaging with results derived from the methodologies discussed opens a window into the larger conversation surrounding plasmids. The roles they play in horizontal gene transfer provide insights that extend into the realms of antibiotic resistance and genetic modification, areas of significant concern in today’s world.
"Plasmids are not just genetic backups; they are key players in bacterial evolution and adaptation, enabling rapid responses to environmental pressures."
Interpretation of Results in the Context of Existing Literature
As existing literature highlighting plasmid functionality is dissected, one can see a pattern: the more we understand plasmids, the better we equip ourselves to tackle pressing issues in health and agriculture. For instance, the use of plasmids in developing genetically modified organisms (GMOs) shows immense potential for improving crop resistance to pests and diseases.
Implications for Future Research or Practical Applications
The implications of plasmid research ripple far and wide. From a practical perspective, both healthcare and agricultural industries stand to gain tremendously. Future research could focus on
- Optimizing plasmid design for gene therapy.
- Understanding plasmid behavior under various environmental conditions.
- Exploring new applications in synthetic biology.
These avenues not only pave the way for innovation but also challenge us to reconsider how we interact with the natural world, reinforcing the importance of plasmids in shaping contemporary science and technology.
As this exploration unfolds, embracing the complexity and significance of plasmids serves as a compass, guiding us through the myriad possibilities that lie ahead.
Prolusion to Plasmids
Plasmids have emerged as essential entities in the realm of microbiology, offering insights into the complexities of genetic material beyond the confines of chromosomal DNA. Their study enriches our understanding of bacterial evolution, resistance mechanisms, and the intricate nature of gene expression. Through this exploration, we uncover myriad applications that have profound implications in fields spanning from biotechnology to medicine and agriculture.
Definition of Plasmids
Plasmids are small, circular DNA molecules distinct from chromosomal DNA. These structures can replicate independently within bacterial cells, effectively functioning as vehicles for genetic information. They often carry genes that confer beneficial traits, such as antibiotic resistance, enabling bacteria to adapt swiftly to environmental changes.
Plasmids are characterized by their versatility. They vary in size, from a few thousand base pairs to over a hundred thousand. Furthermore, they can harbor multiple genes, impacting metabolic processes or traits vital to bacterial survival. This adaptability underscores their importance in microbial genetics and biotechnology.
Historical Context
The discovery of plasmids traces back to the 1950s, but it wasn't until the 1970s that their significance in genetic research started to gain traction. The revolutionary work of Paul Berg and others paved the way for recombinant DNA technology, demonstrating how these elements could be used as vectors to transfer genes across different organisms.
As scientific inquiry advanced, the recognition of plasmids not only grew, but also evolved. These molecular tools reshaped research methodologies, spawning innovations like the first genetically modified organisms (GMOs). The timeline of plasmid research is marked by major breakthroughs that have fundamentally altered our understanding of genetics and paved the way for modern advancements in various fields.
"Plasmids are a testament to the fact that the simplest genetic structures can hold the key to some of the most complex biological functions.”
This historical backdrop sets the stage for a deeper dive into the nuances of plasmid structure, function, and their expansive role in biotechnology and medicine. Understanding the past not only illuminates their current applications but also positions us to explore future innovations in genetic research.
Plasmid Structure
Understanding the structure of plasmids is crucial as it lays the foundation for grasping their diverse functions and applications. Plasmids are not just mere circles of DNA; their intricate composition and variations significantly impact how they interact with host bacteria and the mechanisms through which they replicate and transfer genetic material. This section will dissect the basic constituents of plasmids and shed light on the different types that exist, highlighting their significance in both microbiological research and biotechnological applications.
Basic Composition
Plasmids typically consist of closed, circular double-stranded DNA. They are distinguished from chromosomal DNA by their smaller size and the fact they exist independently of the bacterial chromosome. At their core, plasmids possess essential elements that allow them to function effectively within host cells:
- Origin of Replication (ori): This is where replication initiates, ensuring the plasmid is copied when the cell divides.
- Selectable Markers: These genes confer an advantage, such as antibiotic resistance, allowing researchers to select for cells that have taken up the plasmid.
- Multiple Cloning Site (MCS): This area contains several unique restriction enzyme sites that facilitate the insertion of foreign DNA.
The combination of these elements allows plasmids to be utilized effectively in genetic engineering and biotechnology. The precise structure can vary significantly among different plasmids, influencing their usability in research and therapeutic applications.
Types of Plasmid DNA
Plasmids can be classified into several types, with each holding unique characteristics and functions that make them indispensable in scientific research.
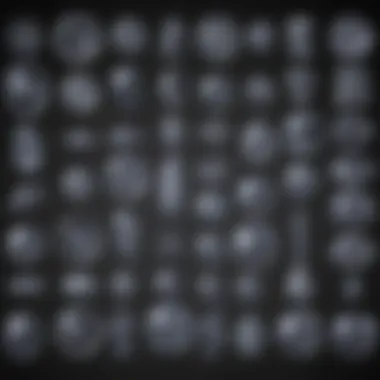
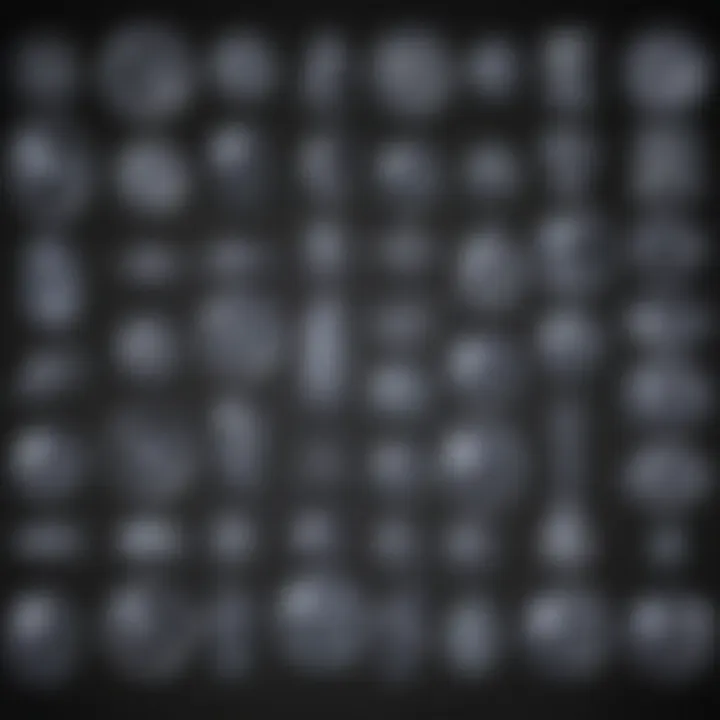
Conjugative Plasmids
Conjugative plasmids are fascinating entities that carry genes necessary for the process of conjugation, a form of horizontal gene transfer. Their most significant contribution is that they enable bacteria to share genetic material directly through physical contact. What sets conjugative plasmids apart is their ability to mobilize their own transfer without requiring other factors.
- Key Characteristic: They carry genes that form the sex pilus, a bridge that allows direct transfer to another bacterial cell.
- Advantages: This capability is vital for spreading traits like antibiotic resistance between bacterial populations, contributing to the rapid evolution observed in many pathogens today.
However, a downside can be their potential to lead to an exponential increase in resistance among bacterial communities, posing significant challenges in treating infections.
Non-conjugative Plasmids
Unlike their conjugative counterparts, non-conjugative plasmids cannot initiate conjugation independently. They often rely on their host bacteria to facilitate transfer via other means, such as transformation or transduction. Despite this limitation, non-conjugative plasmids play unique roles in bacterial ecology and genetic studies.
- Key Characteristic: These plasmids often carry beneficial genes, such as those encoding metabolic pathways or virulence factors.
- Advantages: Due to their inability to spread rapidly on their own, they can serve as quieter genetic tools in research, where control over gene expression is necessary.
However, their dependency on other mechanisms for transfer can make them less flexible in certain experimental settings compared to conjugative plasmids.
Structural Variations
Structural variations within plasmids encompass a wide range of forms that can dramatically affect their function and utility. This aspect is essential as various forms enable plasmids to perform specialized functions.
- Key Characteristic: Plasmids can exist in different forms, such as linear or branched configurations, even though the circular form is most common.
- Advantages: This diversity allows for adaptation to different environments and functions, highlighting the evolutionary strategies of bacteria. Plus, some structurally altered plasmids can have enhanced stability or replication efficiency, which is desirable in synthetic biology applications.
However, these variations also introduce complexities in laboratory settings, where the exact nature of a plasmid can impact experimental outcomes.
Understanding these structures will help researchers tailor their applications and navigate the complex interplay of plasmids and host organisms.
Mechanisms of Plasmid Replication
Understanding the mechanisms of plasmid replication is crucial, providing insights not just into the biology of plasmids themselves but also their practical applications in fields like genetic engineering and biotechnology. These mechanisms are key to how plasmids maintain their presence in bacterial populations, ensuring that important traits, such as antibiotic resistance, can be swiftly exchanged among bacteria.
Replication Processes
Bidirectional Replication
Bidirectional replication involves the simultaneous synthesis of new DNA strands in two directions, starting at a single origin. This approach is characterized by its efficiency, often allowing for faster replication of the plasmid. In essence, as one strand is synthesized in one direction, the other is being synthesized in the opposite direction. Because the process is rapid, bidirectional replication enables bacteria to quickly propagate valuable plasmids through generations.
A key feature of this process is the use of replication forks, which are formed at the origin and spread outwards. This mechanism is popular because it ensures that both daughter cells receive equivalent plasmid copies during cell division, crucial for maintaining the traits that the plasmid carries.
The advantage of bidirectional replication lies in its speed and reliability, especially in conditions where rapid bacterial growth is favored. However, it is not without its caveats, such as potential replication errors or conflicts that may occur if multiple plasmids compete for the same cellular resources.
Unidirectional Replication
In contrast, unidirectional replication is characterized by the synthesis of a new DNA strand in one direction only. This means that, unlike bidirectional replication, it employs a more linear approach to plasmid duplication. Often found in specific plasmids, this technique can contribute to a unique set of challenges and benefits.
The hallmark of unidirectional replication is its simplicity; it requires fewer resources compared to its counterpart. This method is beneficial in low-growth environments where the rapid copying of genetic material is less critical.
However, this type of replication may lead to unbalanced distribution when plasmids are passed on during cell division. Thus, while it provides a straightforward approach, the disadvantage is that it may not be as robust during rapid cell division, potentially leading to loss of plasmid DNA in progeny if not properly managed.
Plasmid Maintenance in Cells
Effective plasmid maintenance is essential for ensuring that plasmids remain stable within bacterial cells. This involves various systems and strategies that bacteria employ to keep plasmids in check, preventing loss during cell division. Primarily, bacteria adapt mechanisms to minimize the likelihood of plasmid loss, including partitioning systems, which help segregate plasmid DNA into both daughter cells during cell division.
In addition to partitioning, plasmids may also utilize maintenance mechanisms, including the action of specific proteins that bind to DNA, ensuring it is replicated and passed on efficiently. These proteins can act as counters to other cellular processes that might inadvertently lead to the loss of plasmid copies.
With these mechanisms in place, plasmids can play a reliable role in microbial ecology, sustaining traits that are vital for survival in a changing environment. It's clear that the interplay of these replication processes and their maintenance reflects on how plasmids contribute to the broader biological narrative.
Plasmid Transfer Mechanisms
The transfer of plasmids is a critical process in microbial genetics, influencing how traits are shared among bacteria. Understanding these mechanisms gives us insight into how genetic material moves and mutates in communities of microorganisms. This section dissects the three primary methods of plasmid transfer: conjugation, transformation, and transduction. Each method offers a distinct pathway for plasmid acquisition, contributing not only to genetic diversity but also to the persistence of certain traits, like antibiotic resistance.
Conjugation
Conjugation is often considered the main event in plasmid transfer, functioning much like a biological handshake between two bacteria. During this process, one bacterium acts as the donor, often referred to as the F+ cell, while the other, known as the recipient or F- cell, receives genetic material. What makes conjugation particularly fascinating is its reliance on contact through a pilus – a thin, tube-like structure that forms between the two bacteria.
- Process of Conjugation:
- Significance:
- The donor bacterium extends its pilus towards the recipient.
- This pilus then retracts, bringing the two cells close enough to establish a bridge.
- DNA is transferred from the donor to the recipient through this bridge, resulting in the recipient acquiring the plasmid.
- This method of transfer is crucial for the spread of antibiotic resistance genes, which can have significant public health implications.
- Conjugation can occur between different bacterial species, thus playing a vital role in genetic diversity and innovation within microbial communities.
Transformation
Transformation refers to the process by which a bacterium takes up free-floating DNA from its environment. This mechanism can be likened to an open-door policy for bacteria – they can snatch up genetic material lying around from lysed cells, allowing them a chance to adapt and survive.
- Mechanism of Transformation:
- Applications and Considerations:
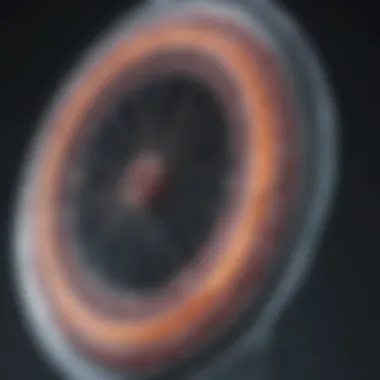
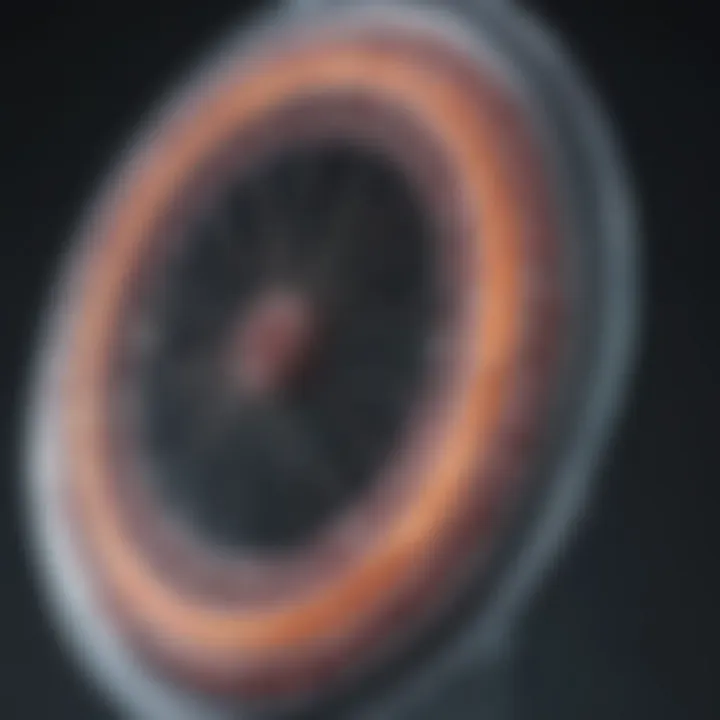
- Competent bacteria, which can naturally take up DNA, do so by integrating it into their genome or by maintaining it as a plasmid.
- This uptake can happen spontaneously, but some bacteria require specific conditions or treatments to become competent.
- Transformation is often harnessed in laboratory settings, especially in genetic engineering, to introduce new traits into bacterial cells.
- This method also raises questions regarding genetic integrity and the unintentional consequences of bringing new traits into existing populations.
Transduction
Transduction is a unique method of DNA transfer mediated by bacteriophages – viruses that infect bacteria. It’s like a genetic courier service where a virus helps to deliver plasmid DNA from one bacterium to another.
- Transduction Process:
- Importance of Transduction:
- During the lytic cycle, a bacteriophage may inadvertently incorporate bacterial DNA, including plasmid DNA, and transfer it when it infects another bacterial cell.
- There are two types of transduction: generalized and specialized. In generalized transduction, any bacterial gene can be packaged; specialized transduction only transfers specific genes associated with the prophage.
- This method promotes genetic variability within bacterial populations and can facilitate the rapid spread of advantageous traits.
- It also plays a role in the evolution of pathogenicity, as new genes entered through transduction can enhance a bacterium's virulence.
Understanding plasmid transfer mechanisms is crucial for harnessing the power of plasmids in biotechnology and medicine.
In summary, the transfer mechanisms of plasmids—conjugation, transformation, and transduction—are foundational processes in microbial genetics that ensure the survival and adaptability of bacterial populations. By exploring these pathways, we uncover the secrets of how bacteria manipulate their genetic material, how they share traits, and how this exchange impacts fields from agriculture to medicine.
Functional Roles of Plasmids in Bacteria
Plasmids play an intriguing role in the survival and adaptability of bacteria. They are extrachromosomal DNA molecules that can confer various advantages to their bacterial hosts. Understanding these functional roles provides essential insights into microbial ecology and the development of antibiotic resistance and metabolic capabilities.
Antibiotic Resistance
One of the most striking roles of plasmids is their involvement in antibiotic resistance. Many bacteria possess plasmids that carry genes, known as resistance genes, which enable them to survive in the presence of antibiotic agents. These genes can encode for proteins that degrade antibiotics, alter their target sites, or pump the drugs out of the cell. As bacteria encounter antibiotics through selective pressures in their environments, they can acquire these plasmids, often through horizontal gene transfer.
- Horizontal Gene Transfer Mechanisms: Plasmids can be shared between bacterial cells through conjugation, a process where two bacteria connect and transfer genetic material. This not only speeds up the spread of resistance genes within a population but can also lead to multi-drug resistance, making infections difficult to treat.
- Epidemiological Impact: The rise of antibiotic-resistant bacteria, fueled by plasmid-mediated gene transfer, poses a substantial challenge for public health. Common examples include Escherichia coli and Staphylococcus aureus, which have developed resistance to multiple antibiotics, largely due to plasmid acquisition.
"The ability of plasmids to confer antibiotic resistance is one of the leading factors complicating treatment options for infectious diseases in our modern medical landscape."
Metabolic Functions
In addition to their role in antibiotic resistance, plasmids significantly contribute to various bacterial metabolic functions. Through plasmids, bacteria can obtain new metabolic pathways which allow them to thrive in specific environments or utilize novel substrates.
- Degradation of Compounds: Some plasmids possess genes that enable bacteria to breakdown environmental pollutants, such as heavy metals or hydrocarbons from oil spills. For instance, certain strains of Pseudomonas species have plasmids that facilitate the degradation of aromatic compounds, thus playing a crucial role in bioremediation efforts.
- Synthesis of Metabolites: Plasmids can also encode genes responsible for producing valuable metabolites. This includes enzymes for biosynthesis, such as those involved in producing secondary metabolites that can exhibit antibiotic or antifungal properties themselves.
- Examples of Metabolic Pathways: Bacteria with specialized plasmids may consume sugars, amino acids, or lipids that would typically be unavailable to their non-plasmid bearing counterparts. This adaptability enhances their survival, especially in nutrient-limited environments.
Understanding these functional roles of plasmids not only sheds light on bacterial evolution but also informs strategies for managing antibiotic resistance and harnessing bacterial capabilities for environmental sustainability.
Plasmids in Genetic Engineering
Genetic engineering stands as a cornerstone of modern biology, and plasmids play an indispensable role in this realm. They are not just carrying bits of genetic code; these mobile genetic elements have become the workhorse of biotechnology, enabling scientists to manipulate the genetic material in organisms with pinpoint accuracy. When it comes to thinking about plasmids, one could say they're like the Swiss Army knife of genetic engineering – versatile and endlessly useful.
Vectors for DNA Cloning
Plasmids are primarily used as vectors in DNA cloning. This means they carry foreign DNA into a host cell, allowing for the replication and expression of that DNA. To achieve this, plasmids usually contain essential elements that facilitate cloning, such as:
- Origin of replication (ori): Ensures that the plasmid can replicate independently within the host.
- Multiple cloning site (MCS): A segment with several restriction enzyme sites for inserting foreign DNA.
- Selectable marker: Usually an antibiotic resistance gene that helps in identifying the cells that have taken up the plasmid.
Take, for instance, the usage of the pBR322 plasmid, which has been foundational in cloning practices. By inserting a gene of interest into its MCS, scientists can create clones of the target gene in bacteria, allowing for large-scale production. It’s like having a key that unlocks an entire treasure trove of possibilities in the genetic landscape.
However, while plasmids offer tremendous benefits, there are considerations. Not all plasmids behave the same way in all host cells, and the efficiency of transformation can vary. Thus, selecting the right plasmid is crucial; the choice impacts everything from the yield of the gene product to the stability of the expression.
Gene Therapy Applications
Gene therapy is another area where plasmids make their mark. This technique involves delivering therapeutic genes to patients in order to treat or even cure diseases. Plasmids utilized in gene therapy can carry a functional copy of a gene to replace a faulty one, potentially reversing the symptoms of genetic disorders.
In practical terms, for conditions such as cystic fibrosis or hemophilia, plasmids provide a means of delivering genes safely. Researchers deploy specific plasmids designed to target particular cells in the human body, aiming for those cells to incorporate the plasmid and express the therapeutic protein. This tailoring process enhances the efficacy of treatment and minimizes side effects, showcasing the advanced thinking behind the application of plasmids.
Nonetheless, gene therapy is not without its challenges. The delivery method must ensure that the plasmid reaches its target cells effectively. Furthermore, there's a need to monitor for any adverse reactions, such as unintended immune responses. Therefore, while great strides have been made, the landscape of plasmid-based gene therapy continues to evolve, driven by ongoing research and a greater understanding of genetic mechanisms.
"In the race against genetic diseases, plasmids represent not just a tool but a beacon of hope for many patients."
Through elucidating both vector and therapy roles, we start to grasp why plasmids are so vital in genetic engineering – they are crucial to transforming theoretical science into practical, groundbreaking applications.
Plasmids in Biotechnology
The role of plasmids in biotechnology is multifaceted and indispensable. They serve as key tools in manipulating genetic material and driving advancements in various fields, particularly agriculture and medicine. Plasmids allow scientists and researchers to alter the genetic makeup of organisms, leading to significant innovations and solutions to pressing global challenges. This section will shed light on how plasmids are leveraged in biotechnology, emphasizing their applications in agriculture and industry.
Utilization in Agriculture
Agriculture has seen a profound transformation due to the incorporation of plasmids into plant genetics, most notably in the creation of genetically modified organisms (GMOs) and pest-resistant crops. These developments not only aim to improve yield but also to ensure food security in an ever-growing world population.
Genetically Modified Organisms
Genetically modified organisms are created by introducing specific genes into a plant’s genome using plasmids as vectors. This technology allows for the precise modification of plants to express desired traits, such as enhanced nutritional content or drought resistance. GMOs have a key characteristic: they can be engineered to withstand harsh environmental conditions, thus reducing the reliance on chemical fertilizers or pesticides. This botanical bravado comes with distinct advantages, like increased agricultural productivity and the potential for reducing biodiversity loss caused by traditional farming methods.
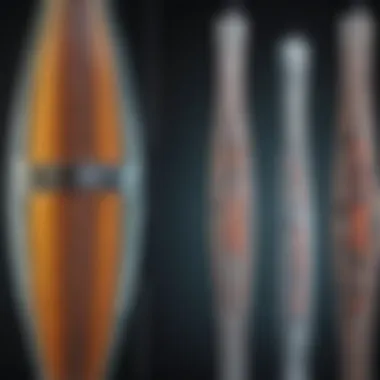
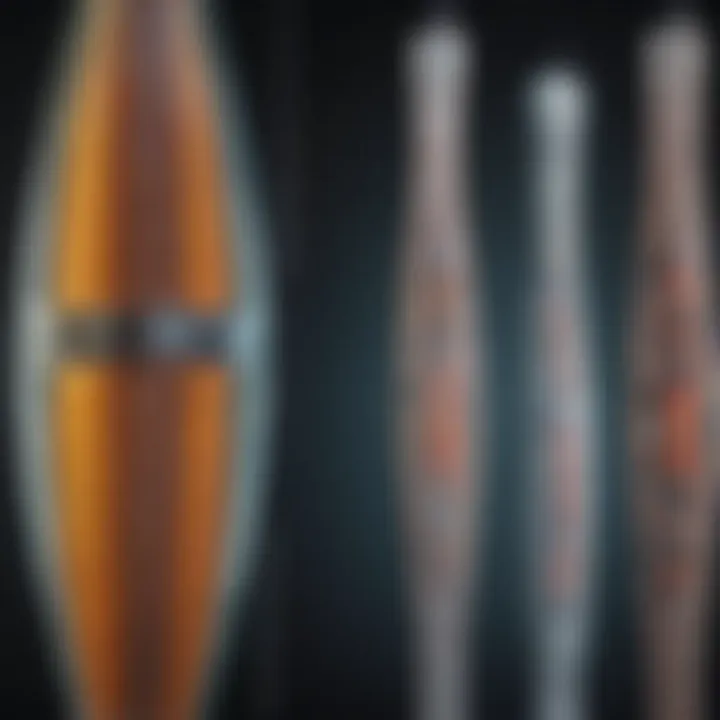
One unique feature of GMOs is their ability to produce plant varieties that can thrive in less-than-ideal soil conditions, or can endure pest pressures without the need for additional chemicals. However, there are also notable disadvantages that accompany GMOs, such as public resistance based on safety concerns and ethical dilemmas surrounding biodiversity.
Pest Resistance
Pest resistance is another crucial application enabled by plasmids. This aspect of biotechnology allows for the development of crops that can naturally deter pests, significantly lowering the damage that insects can inflict on plants. By inserting genes from naturally pest-resistant species into crops, scientists create plants that exhibit built-in defenses against specific pests. This offers a sustainable alternative when compared to chemical pesticides, which can have harmful effects on both the environment and human health.
The key characteristic of pest-resistant crops is their ability to produce proteins that target and neutralize specific insect species. This trait makes them especially popular among farmers looking to minimize agricultural loss while also adhering to environmentally friendly practices.
Nevertheless, pest resistance comes with its own set of challenges. Over time, pests may develop resistance to these genetically engineered defenses, resulting in a potential resurgence of pest populations that could negate the advantages of biotechnologically modified crops.
Industrial Applications
When we shift our focus from agriculture, we see that the industrial sector has also reaped substantial benefits from plasmids. Plasmids are instrumental in the production of enzymes, biofuels, and pharmaceuticals. For instance, genetically engineered bacteria can be employed to produce enzymes that are essential in the textile, food, and detergent industries.
Furthermore, in the realm of biofuels, plasmids facilitate the creation of microbial strains capable of converting waste biomass into renewable energy sources, which aligns with global efforts to reduce carbon footprints. The pharmaceutical industry also thrives on plasmid technologies; plasmids are used to develop vaccines and therapeutic agents, revolutionizing how we combat diseases.
The ethical considerations and regulatory frameworks surrounding these applications play a critical role. Encouragingly, the collaborative efforts among scientists, regulators, and industry stakeholders aim to strike a balance between innovation and safety, ensuring that the advancements made through plasmid technology benefit society as a whole.
"Plasmids have transformed biotechnology into a powerful ally in addressing some of the most pressing challenges we face today."
Challenges and Ethical Considerations
When we dive into the fascinating world of plasmids, it’s not all smooth sailing. Understanding the challenges and ethical considerations surrounding plasmids becomes imperative. This section seeks to shine a light on significant aspects influencing research and application of plasmids in our society today. From the risk of horizontal gene transfer to the regulatory frameworks in place to mitigate these risks, comprehending these elements helps to ensure responsible science.
Risk of Horizontal Gene Transfer
One of the most pressing concerns related to plasmids is the risk of horizontal gene transfer (HGT). This process allows genetic material to be transferred between organisms in a manner that doesn’t involve the typical parent-to-offspring inheritance. In simpler terms, it’s like passing notes in class but with genetic information. While this phenomenon can be beneficial, such as when it grants bacteria antibiotic resistance, it can also lead to unexpected consequences.
Specifically, the implications of HGT in medicine and agriculture can’t be overlooked:
- Antibiotic Resistance: Bacteria capable of sharing resistance genes via plasmids pose a significant threat to public health. The frequent use of antibiotics can select for these resistant strains, making infections harder to treat.
- Gene Spread in Environment: In agricultural applications, genetically modified organisms (GMOs) could inadvertently transfer their traits to wild relatives. This could lead to ecological imbalances or the emergence of super-weeds.
In this vein, researchers must tread carefully when working with plasmids, balancing innovation with caution. The mishaps of the past serve as a reminder of the potential dangers lurking in genetic manipulation.
"With great power comes great responsibility." - A timeless adage that resonates deeply in the scientific field, especially when it comes to genetic engineering.
Regulatory Frameworks
The dance between technological advancements and ethical considerations comes alive through regulatory frameworks. These frameworks are designed to mitigate risks associated with plasmid research and applications. Countries and international bodies have established regulations to guide the safe use of plasmids, ensuring that their beneficial uses do not come at the cost of public or environmental safety.
Essential elements of these frameworks include:
- Assessment Procedures: Before a plasmid-containing organism can be released into the environment, thorough evaluation is critical. This process may involve risk assessments to evaluate potential impacts on ecosystems or human health.
- Public Consultation: Engaging with the community is vital. Stakeholders, including local populations, have the right to voice concerns about potential risks related to GMO crops or clinical applications of plasmids in gene therapies.
- International Guidelines: Bodies like the World Health Organization and Food and Agriculture Organization provide extensive guidelines that help shape national regulations. These guidelines ensure a consistency in the handling and understanding of plasmids across borders.
As we move forward in this exciting yet uncertain realm, the integration of ethical considerations and robust regulatory frameworks will play a crucial role. The balance between scientific exploration and ethical accountability isn't just necessary; it’s vital for a sustainable future in genetic research.
Future Directions in Plasmid Research
The field of plasmid research is on the cusp of exciting developments that could reshape our understanding and application of these vital elements in molecular biology. As researchers plunge deeper into the capabilities and variations of plasmids, several future directions emerge, dovetailing advancements in synthetic biology and therapeutic applications. These discussions are not merely academic; they possess the potential for significant real-world impacts on medicine, agriculture, and environmental science.
Synthetic Biology Advances
Synthetic biology stands as a fertile ground for the evolution of plasmid research. The toolbox offered by synthetic biology enables scientists to design and construct new biological parts, devices, and systems. By leveraging plasmids as vectors, researchers can engineer bacteria to perform novel functions—ranging from biofuel production to biosensing capabilities.
One major advancement is in the realm of optimized plasmids, designed to enhance stability and expression in host cells. By studying how plasmids interact with cellular machinery through various environmental conditions, synthetic biologists can create custom plasmids that achieve a higher level of efficiency. Furthermore, synthetic pathways using plasmids can be developed to boost the production of valuable compounds. Recent projects have even aimed at creating multi-functional plasmids capable of performing several tasks simultaneously.
The concept of modular plasmids also gains traction. These plasmids could be assembled in such a way that researchers can swap out functional components as needed. This flexibility could lead to rapid iterations in experimental designs, making the process of genetic engineering much more nimble and innovative.
Innovations in Therapeutic Applications
On the therapeutic front, plasmids are increasingly being recognized for their role in gene therapy. As understanding of the human genome expands, so too does the idea of using plasmids to deliver genes that can correct genetic defects or introduce new functions to cells. This approach shows promise in treating a range of genetic disorders, from muscular dystrophy to certain forms of cancer.
One fascinating development involves the use of plasmids to formulate personalized medicine treatments. With the ability to tailor plasmid design based on a patient's specific genetic makeup, researchers hope to enhance the effectiveness of targeted therapies. For example, a customized plasmid could deliver a therapeutic gene that addresses the unique genetic anomaly present in a patient's tumor cells.
Moreover, advancements in delivery systems are crucial for the success of these therapies. Researchers are exploring nanoparticle-based delivery, electrotransfection methods, and even viral vectors, in which plasmids are packaged within a virus to facilitate uptake by the host cells. Each of these methods has advantages and requires careful consideration of safety and efficacy.
Ending
Understanding plasmids is crucial in today's scientific landscape. The multifaceted roles these tiny circular DNA molecules play extend well beyond the confines of microbiology. In the context of this article, we have explored various aspects, from their structural characteristics to their function in genetic engineering and biotechnology applications. Recognizing the importance of plasmids allows for a better grasp of genetic manipulation techniques that have broad implications in agriculture, medicine, and environmental processes.
Summary of Key Points
- Structure and Composition: Plasmids are composed of DNA and can vary significantly in size and type. The basic elements include genes responsible for specific functions and sequences that facilitate replication and transfer.
- Modes of Replication: They replicate independently within bacterial cells, employing mechanisms such as bidirectional and unidirectional replication to maintain their existence.
- Transfer Mechanisms: Methods such as conjugation, transformation, and transduction highlight the ability of plasmids to share genetic material across different organisms.
- Functional Roles: They are pivotal in antibiotic resistance and unique metabolic functions, shaping bacterial survival strategies.
- Applications in Biotechnology: Plasmids serve as essential tools in genetic engineering, used as vectors in DNA cloning and in developing gene therapy strategies.
- Ethical Considerations: The potential for horizontal gene transfer raises important ethical questions, necessitating thorough regulation and oversight to mitigate risks.
Implications for the Future of Science
The future of plasmid research holds tremendous potential. As synthetic biology advances, researchers may develop novel plasmids engineered for specific applications. This could improve processes in several sectors:
- Medicine: Enhanced plasmids could lead to effective gene therapies, potentially treating genetic disorders that have long been considered untreatable.
- Agriculture: Genetically modified organisms that incorporate plasmids may provide solutions for pest resistance, reducing reliance on chemical pesticides.
- Industrial Uses: Innovations in using plasmids in bioproduction could revolutionize the manufacturing processes for biofuels and biodegradable plastics.
Plasmids aren't just passengers in bacterial cells; they are the keys to unlocking the future of genetic applications.
Ultimately, fostering a critical understanding of plasmids not only benefits the scientific community but also informs ethical debates surrounding genetic engineering's applications in society. As we continue to unlock the mysteries of these structures, their potential influence on life, science, and technology becomes increasingly apparent, paving the way for groundbreaking advancements.