Understanding Sickle Cell Disease and Its Causes
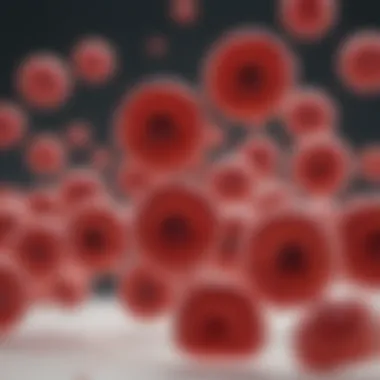
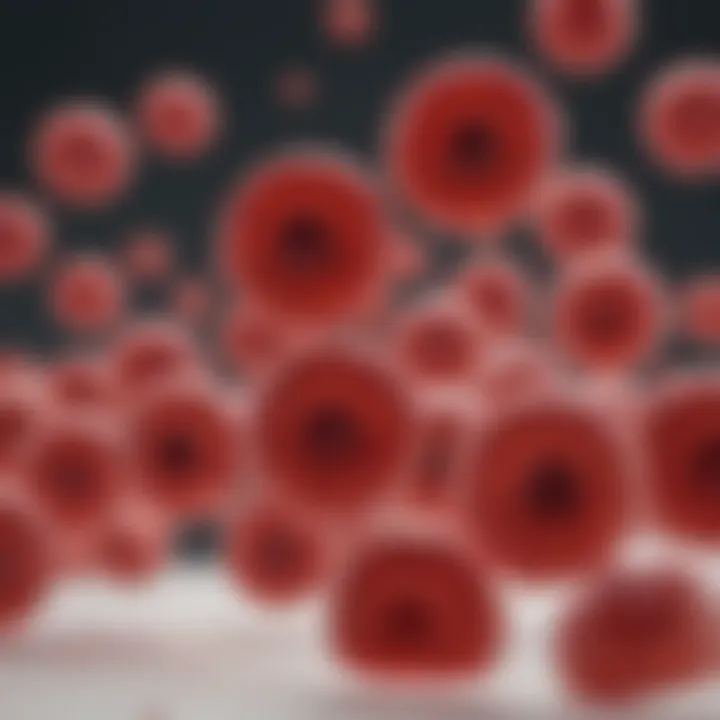
Intro
Sickle cell disease, characterized by the presence of sickle-shaped red blood cells, poses significant health challenges worldwide. Understanding the underlying causes of this condition is essential, not just for treatment, but for preventive strategies as well. The formation of these abnormal cells can be traced to a complex interplay of genetic, molecular, and environmental factors. This article aims to delve deeply into these aspects, shining a light on why and how red blood cells take on this abnormal shape.
The Significance of Sickle-Shaped Red Blood Cells
Sickle-shaped red blood cells are not merely an interesting biological anomaly; their presence significantly impacts oxygen transport and blood flow within the body. These cells can clump together, leading to blockages in small blood vessels. This results in pain and potential damage to organs and tissues. Hence, a comprehensive understanding of the causes of their formation serves not only academic interest but also profound implications for patient care and management strategies.
Key Points to Explore
- Genetic Factors: The blueprint of an individual's cells is found in their genes. In the case of sickle cell disease, mutations in the HBB gene play a crucial role.
- Molecular Mechanisms: Specifically, the structure of hemoglobin is directly implicated in the sickling processes. Changes in its makeup can drastically alter how red blood cells function.
- Environmental Influences: Various physiological conditions, such as low oxygen levels or dehydration, can potentiate sickling episodes.
Overall, the discussion will unravel how these components work together to foster the characteristic sickle shape of red blood cells, ultimately enhancing our awareness of the complex biology behind this hematological condition.
Prolusion to Sickle Cell Disease
Sickle cell disease (SCD) presents a unique challenge in the realm of hematology. It’s a condition that affects millions worldwide, significantly altering the quality of life for those diagnosed with it. Understanding the intricacies of this disease is crucial not just for healthcare professionals but also for patients and their families grappling with its realities. This section aims to lay the groundwork for our discussion by highlighting key elements of the disease, its implications, and why this exploration is critical.
Overview of Red Blood Cell Function
Red blood cells (RBCs) serve a vital role in the human body. Their primary function is to transport oxygen from the lungs to the tissues and take carbon dioxide back for exhalation. The standard RBC is disc-shaped and flexible, allowing it to navigate through the body's narrow capillaries without issue. This flexibility is essential for maintaining healthy circulation and ensuring that every cell receives the oxygen it needs.
The typical life span of an RBC is about 120 days. After which, they are broken down and their components recycled to produce new blood cells. However, in the case of sickle cell disease, the shape and integrity of these cells are compromised due to a genetic alteration in hemoglobin, the protein responsible for oxygen transport.
Definition of Sickle Cell Disease
Sickle cell disease is an inherited blood disorder characterized by the presence of abnormal hemoglobin, termed hemoglobin S. This abnormal hemoglobin can cause red blood cells to deform into a rigid, sickle-like shape, especially under low oxygen conditions. Unlike normal RBCs, these sickle-shaped cells can become stuck in blood vessels, impeding blood flow and leading to pain, organ damage, and other serious complications.
The genetic mutation responsible for this condition occurs in the HBB gene, which codes for the beta-globin subunit of hemoglobin. A person inherits two copies of the mutated gene—one from each parent—to be classified with sickle cell disease. Alternatively, inheriting just one copy leads to sickle cell trait, typically resulting in milder symptoms but also carrying the risk of passing on the condition to future generations.
This condition presents a variety of symptoms ranging from chronic pain episodes, known as crises, to more severe complications like stroke or acute chest syndrome. Sickle cell disease is not just a medical concern; it encompasses socioeconomic factors, mental health issues, and significant lifestyle impacts, hence the need for a thorough understanding of its underlying causes.
Understanding sickle cell disease is not merely an academic exercise but a pathway to improvement in the lives of those affected.
As we delve deeper into the genetic, biochemical, and environmental factors influencing the sickle shape of red blood cells, we set the stage for increasing our collective grasp of this complex and multifaceted disease.
Anatomy of Normal Red Blood Cells
The anatomy of normal red blood cells is crucial in understanding how these cells function and how their structure can lead to abnormalities like the sickle shape. Normal red blood cells, also known as erythrocytes, primarily serve the essential role of transporting oxygen from the lungs to the body’s tissues and bringing carbon dioxide back for exhalation. By exploring the specific elements that contribute to the anatomy of these cells, we gain insight not only into their normal functions but also how deviations in their structure can result in clinical conditions such as sickle cell disease.
Shape and Flexibility
Normally, red blood cells have a biconcave disc shape, which is often likened to a doughnut without a hole. This unique design offers several benefits:
- Increased Surface Area: The biconcave shape enhances the surface area available for gas exchange, ensuring maximum absorption of oxygen and release of carbon dioxide.
- Flexibility: The flexibility of these cells allows them to navigate through the narrowest capillaries, delivering oxygen efficiently. This is achieved due to the thinness of the cell membrane and the internal structure that permits slight deformation.
When conditions are ideal, red blood cells maintain their shape while also proving their capacity to stretch and fold without breaking. However, numerous factors, such as genetic mutations or changes in environmental conditions, can compromise this flexibility, making them rigid and prone to sickling. The significance here cannot be emphasized enough: it is this very gentleness of shape that facilitates their function; any deviation can severely impair survival rates in the blood stream.
Hemoglobin Structure and Function
At the core of red blood cells’ functionality is hemoglobin, the protein responsible for carrying oxygen. Normal red blood cells are packed with this protein, which is made up of four polypeptide chains, each bound to a heme group. The heme groups contain iron, which is vital for oxygen binding. Here are some key points about hemoglobin structure and its function:
- Oxygen Transport: Each hemoglobin molecule can bind four molecules of oxygen, which is crucial for delivering oxygen to the tissues.
- Carbon Dioxide Transport: Hemoglobin is also involved in transporting carbon dioxide back to the lungs for expulsion.
- pH Buffering: Hemoglobin acts as a buffer, helping to maintain blood pH by binding to hydrogen ions when certain biochemical processes generate excess acidity.
In normal conditions, the interaction between oxygen, carbon dioxide, and hemoglobin occurs seamlessly, ensuring that red blood cells efficiently perform their job. This stability in hemoglobin structure is what prevents the occurrence of diseases like sickle cell disease. However, mutations in the HBB gene alter hemoglobin's properties, promoting abnormal interactions that lead to the formation of sickle-shaped cells.
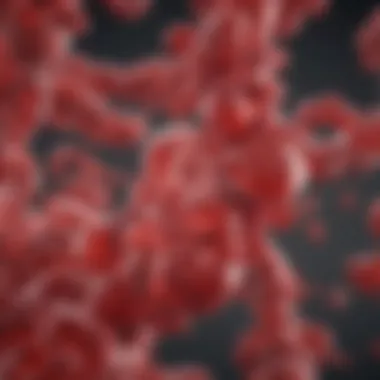
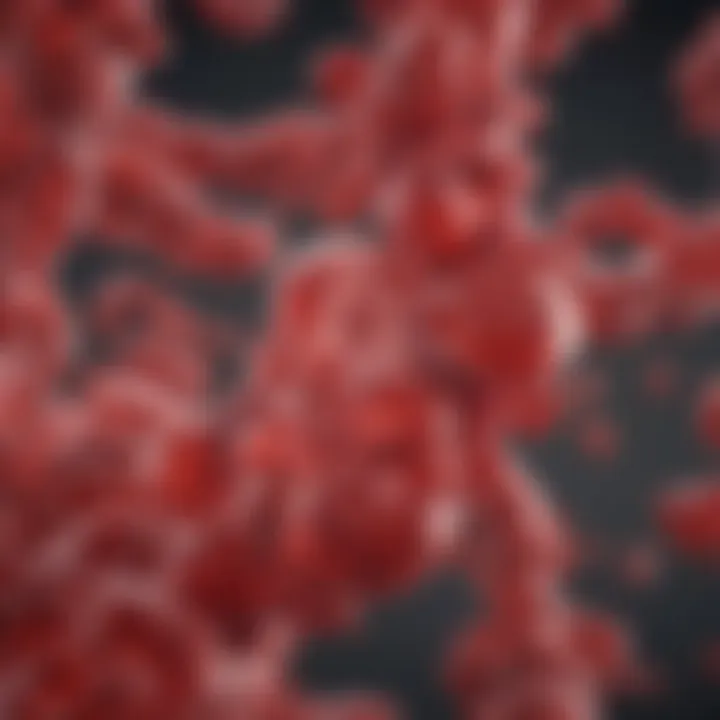
Understanding the anatomy of normal red blood cells not only illuminates the healthy state but also provides a foundation for comprehending the pathological changes that give rise to conditions like sickle cell disease.
Genetic Basis of Sickle Cell Disease
The genetic underpinnings of sickle cell disease are fundamental to understanding its prevalence and impact on those affected. This segment of the article lays bare the intricacies of how mutations in a specific gene lead to the formation of sickle-shaped red blood cells, a hallmark of the condition. The focus here is not merely on the existence of the HBB gene but on how variations within it propagate a series of biochemical disruptions. Grasping the genetic framework assists in grasping the implications of inheritance, therapy, and potential future treatments. So, buckle up as we embark on this genetic exploration.
Prelims to the HBB Gene
At the heart of sickle cell disease lies the HBB gene. This gene, situated on chromosome 11, encodes the beta chain of hemoglobin. Hemoglobin is the protein responsible for oxygen transport in red blood cells. Any alteration, or mutation, in this gene can lead to improper hemoglobin formation. Particularly noteworthy is the point mutation that results in hemoglobin S (HbS) instead of normal hemoglobin A (HbA).
As HbS accumulates under low oxygen conditions, the red blood cells morph from their typical doughnut shape into that sickle configuration. The implications of the HBB gene extend beyond mere biochemical changes; they set the stage for the clinical manifestations of the disease.
Mechanisms of Genetic Mutation
When we delve into the mechanisms behind genetic mutations leading to sickle cell disease, we are not just examining a trivial clump of genetic material; we are literally unraveling the thread of fate woven by DNA. The most common mutation associated with sickle cell disease is a substitution of adenine with thymine at the sixth codon of the HBB gene. This seemingly minuscule change encodes a different amino acid—valine instead of glutamic acid—transforming the way hemoglobin behaves under stress.
"A single mutation can create vast ripples in the biological lake that influence health and wellbeing."
As a consequence, in situations of stress, such as dehydration or low oxygen levels, the abnormal hemoglobin aggregates, causing the red blood cells to adopt that characteristic sickle shape.
Inheritance Patterns of Sickle Cell Disease
Sickle cell disease follows an autosomal recessive inheritance pattern. This means that an individual must inherit two copies of the mutated HBB gene—one from each parent—to manifest the disease. If a person receives only one mutated gene and one normal gene, they become a carrier, often labeled as having sickle cell trait. These carriers typically do not experience symptoms, yet they can pass the mutated gene on to their offspring.
The statistical chances of having an affected child can be surprisingly significant. For instance, if both parents are carriers, each child has a 25% chance of being affected, a 50% chance of being a carrier, and a 25% chance of having normal hemoglobin.
Understanding these inheritance patterns allows for better family planning and can raise awareness in communities where sickle cell disease is prevalent. It’s like shining a flashlight down a dark path, showing how critical knowledge of genetic transmission can illuminate the risk factors involved.
Biochemical Changes Leading to Sickle Shape
Understanding the biochemical changes that lead to sickle-shaped red blood cells is crucial for unraveling the complexities of sickle cell disease. These changes aren’t just mere details; they play a major role in the symptoms and complications associated with this condition. The formation of sickle shapes in red blood cells is entirely tied to the alterations in the structure and behavior of hemoglobin, the molecule responsible for oxygen transport in the body. By exploring these underlying biochemical phenomena, we can better comprehend how sickle cell disease manifests and affects individuals.
Role of Hemoglobin S
Hemoglobin S is the primary player when it comes to sickle cell formation. This variant of hemoglobin arises from a specific mutation in the HBB gene, which codes for the beta chain of hemoglobin. Unlike normal hemoglobin A, which consists of two alpha and two beta chains, hemoglobin S has a substitution of valine for glutamic acid at the sixth position of the beta globin chain.
When hemoglobin S releases oxygen, it tends to polymerize and form long, rigid structures, thereby causing red blood cells to take on a crescent or sickle shape instead of the usual flexible disc shape. This stickiness and the associated stiffening of the cells can lead to blockages in small blood vessels, restricting blood flow and causing pain crises among other symptoms. Each of these processes builds upon the biochemical characteristics of hemoglobin S itself.
"The sickle shape not only disrupts normal circulation but also amplifies the risk of various complications, such as infections and organ damage."
Aggregation of Deoxygenated Hemoglobin
The aggregation of deoxygenated hemoglobin plays a pivotal role in the sickling phenomenon. When hemoglobin S encounters low oxygen levels—like in areas of increased cellular demand—this variant becomes even more prone to aggregation. When hemoglobin is deoxygenated, the molecular structure shifts, increasing the likelihood of hemoglobin S molecules attracting each other. They form polymers that ultimately distort the cell's shape.
As these polymers grow, the interactions between hemoglobin S molecules create a sort of scaffolding, solidifying the changes that take place in the red blood cell. This alteration severely limits the cell's ability to traverse through the microvasculature. Consequently, the sickle-shaped cells are more fragile and prone to hemolysis, leading to anemia—a significant condition that comes with various health challenges.
The biochemical mechanics of sickling emphasize the importance of oxygen levels in managing sickle cell disease. For instance, during physical exertion or in environments where oxygen saturation is low, individuals with sickle cell disease are at greater risk of sickling due to increased hemoglobin S aggregation. Understanding these mechanisms is paramount for healthcare providers in developing strategies for intervention and treatment.
Physiological Factors Contributing to Abnormal Red Blood Cell Shapes
Understanding how physiological factors contribute to the formation of abnormal red blood cell shapes helps paint a fuller picture of sickle cell disease. It’s not just about genetics or biochemistry; the body’s varied internal conditions can also play a pivotal role. Blood flow and oxygen levels, alongside acidity, are essential elements influencing how blood cells behave in the bloodstream. A thorough grasp of these factors could potentially lead to better management strategies for those affected by sickle cell disease.
Oxygen Levels and Blood Flow
In normal circumstances, red blood cells are quite adept at transporting oxygen. However, in sickle cell disease, the levels of oxygen in the blood can significantly affect the shape of these cells. When oxygen levels drop, hemoglobin molecules present in the red blood cells undergo a transformation. Instead of maintaining their usual flexible and round configuration, these cells can buckle and form a sickle shape.
- Decreased Oxygen Supply: A reduced oxygen environment can occur during physical exertion, extreme temperatures, or even high altitude. When the body is in need of more oxygen, the demand on blood cells increases, compelling them to adapt—not always for the best.
- Blood Flow Dynamics: Blood not flowing smoothly can lead to clumping of the sickle cells, exacerbating tissue hypoxia. This can cause painful crises, where the sickle-shaped red blood cells block blood flow, causing intense discomfort and potential tissue damage.
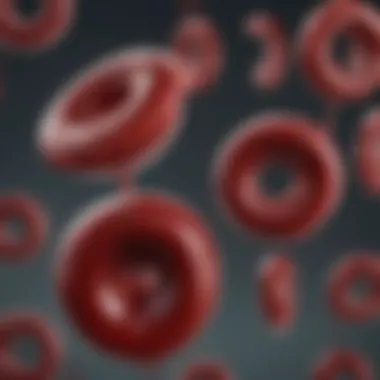
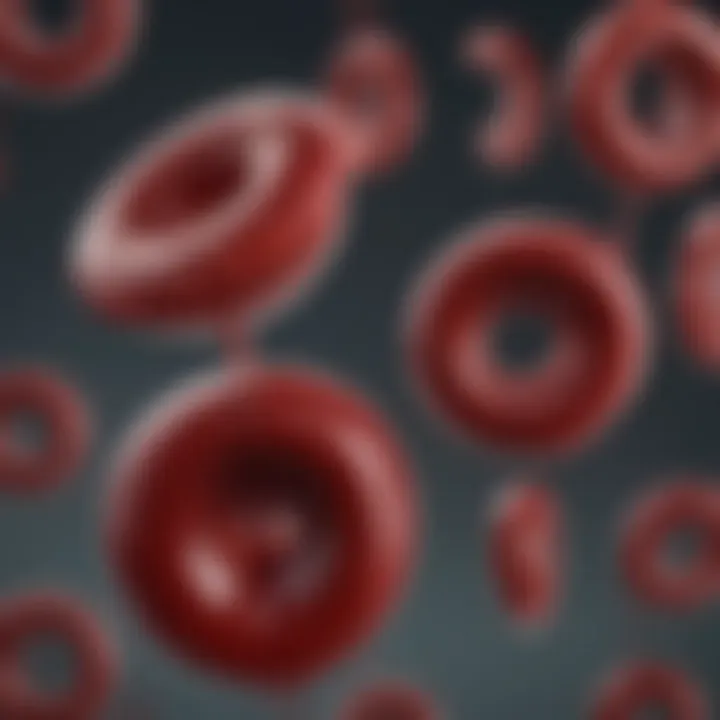
Maintaining optimal oxygen levels is key to ensuring that red blood cells retain their healthy shape. Any interference, whether from external conditions or body demands, can lead red blood cells down a potentially harmful path.
Acidity and Its Effects on Hemoglobin
The acidity of blood, measured by pH levels, plays a crucial role in the stability of hemoglobin. As the pH decreases—meaning the blood becomes more acidic—it affects the hemoglobin's ability to hold and release oxygen efficiently. In more acidic conditions, hemoglobin S, the mutated form present in sickle cell disease, tends to aggregate more easily.
- Proton Concentration: An increase in protons can shift hemoglobin’s configuration, encouraging the formation of chains that lead to sickling. This chain aggregation not only alters red blood cell shapes but also drastically reduces their flexibility.
- Shift in the Oxygen-Hemoglobin Disassociation Curve: A more acidic environment causes hemoglobin to release oxygen more readily, but this can also lead to further sickling. Any situation that results in acidosis in the body can escalate the problems faced by individuals with sickle cell disease.
"Understanding the physiological backdrop allows us to appreciate how lifestyle and environmental factors intertwine with the genetic predisposition to sickling."
Integrating these physiological perspectives gives researchers and healthcare professionals a better understanding of how to improve care strategies and lifestyle recommendations for individuals living with sickle cell disease. It's a call to action to consider how real-life situations, everyday conditions, and even minor changes can have substantial impacts on the health outcomes of those with abnormal red blood cell shapes.
Environmental Influences
The environment plays a pivotal role in the behavior and health of red blood cells, particularly when it comes to sickle-shaped red blood cells. Understanding these influences is crucial, as they can exacerbate or alleviate the symptoms and complications of sickle cell disease. It’s not just about genetics or the molecular complexities; environmental factors can significantly sway how the disease manifests in individuals.
Temperature Effects on Hemoglobin Properties
Temperature is a game-changer when it comes to hemoglobin properties. In a nutshell, higher temperatures tend to reduce the solubility of hemoglobin, leading to increased sickling. When hemoglobin S aggregates due to heat, red blood cells lose their pliability. This loss of flexibility can cause blockage in small blood vessels, resulting in pain and potentially serious complications. Conversely, cooler temperatures can have a stabilizing effect on hemoglobin, enhancing its solubility and thereby helping to keep cells in a more normal shape.
Research has shown that patients living in warmer climates may experience more frequent sickle cell crises, as the heat can trigger deoxygenation of hemoglobin more readily. It’s like cooking; if the pot gets too hot, the ingredients lose their intended form. Gradually understanding these temperature dynamics can lead to better management strategies for people with sickle cell disease.
"Control over environmental factors, such as temperature, might just be the key to managing sickle cell complications effectively."
Impact of High Altitude
High altitude presents its own set of challenges for individuals with sickle cell disease. Reduced oxygen levels at elevated altitudes can lead to hypoxia, which intensifies the risk of hemoglobin S molecules precipitating into a sickle shape. In layman terms, when oxygen is low, it’s like depriving the hemoglobin of its lifeblood, causing it to morph into that troublesome sickle form. Individuals with sickle cell disease living or traveling to higher altitudes are often advised to take precautions, such as increasing fluid intake and ensuring adequate oxygen supply.
Studies indicate that even short trips to high altitudes can increase the risk of vaso-occlusive crises for patients already predisposed to complications. This is primarily because the sickled cells tend to clump together, leading to blockages that can cause severe pain.
Assessing the impact of high altitude can offer valuable insight for health professionals and families affected by sickle cell disease. Understanding these external pressures is just as important as recognizing the internal mechanisms at play.
Clinical Implications of Sickle Cell Formation
Understanding the clinical implications of sickle cell formation is crucial for both patients and healthcare providers in managing the disease effectively. The distorted shape of red blood cells fundamentally alters their behavior, leading to numerous health challenges. Moreover, recognizing these implications helps anticipate complications and tailor appropriate treatment strategies. This section will elucidate the symptoms and complications that arise from sickle cell disease, as well as the diagnostic and screening methodologies employed to identify the condition before significant damage occurs.
Symptoms and Complications
Patients with sickle cell disease often experience a variety of symptoms due to the sickling of red blood cells. One common symptom is pain crises, which occur when sickle-shaped cells block blood flow in small blood vessels, causing severe pain in the chest, abdomen, and joints. This painful episode is often referred to as a “sickle cell crisis.”
Other notable symptoms include:
- Anemia: The sickled cells have a shorter lifespan than normal cells, leading to a constant shortage of healthy red blood cells.
- Fatigue: Due to decreased oxygen delivery to tissues, individuals frequently feel tired and weak.
- Swelling: Particularly in hands and feet, caused by blocked blood circulation.
- Frequent Infections: Sickle cells can damage the spleen, making patients more susceptible to infections.
- Delayed Growth: In children and adolescents, sickle cell disease can impair growth and development due to anemia and nutrient deficiencies.
Complications may range from acute to chronic conditions, such as stroke, organ damage, or lung-related issues like pulmonary hypertension. Each of these complications can drastically impact a patient's quality of life, further emphasizing the importance of monitoring symptoms closely.
"The sickle-shaped red blood cells behave in ways that can greatly influence outcomes, rendering constant attention and proactive management a necessity."
Diagnosis and Screening Methods
Diagnosing sickle cell disease involves a combination of physical examinations and laboratory tests. The following methods are commonly employed:
- Blood Tests: The most definitive test is hemoglobin electrophoresis, which separates different types of hemoglobin, allowing for the identification of Hemoglobin S that characterizes sickle cell disease.
- Complete Blood Count (CBC): This test measures the levels of red blood cells and hemoglobin, helping to assess anemia severity.
- Newborn Screening: Many countries have implemented routine testing for sickle cell disease shortly after birth, allowing for early diagnosis and intervention.
- Genetic Testing: In families with a history of sickle cell disease, genetic testing can be a proactive measure to identify carriers of the sickle cell trait.
By utilizing these methods, healthcare professionals can provide timely treatment options, which may include pain management, vaccination to prevent infections, or regular blood transfusions to manage symptoms and reduce the risk of complications.
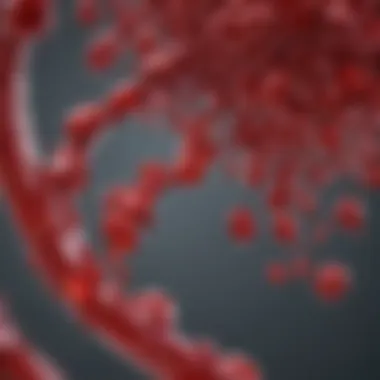
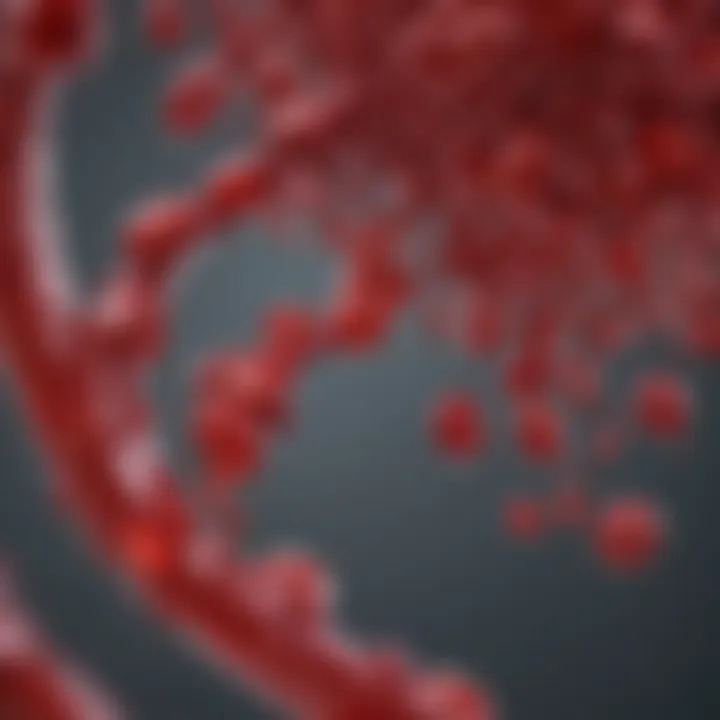
The clinical implications of sickle cell formation are significant and multifaceted. By understanding these aspects, medical practitioners can improve patient management and care, ultimately enhancing quality of life for those with sickle cell disease.
Management and Treatment Options
Understanding the management and treatment options available for sickle cell disease is crucial as it directly impacts the quality of life for those affected. Optimizing treatment can reduce complications, lessen pain crises, and improve overall health outcomes. Here's a close look at some key strategies that have emerged through research and clinical practice.
Transfusion and Hydroxyurea Therapy
Transfusion therapy plays an important role in the management of sickle cell disease. Regular blood transfusions can help to increase the number of normal red blood cells in circulation, thus reducing sickling and the associated complications. In practice, patients often receive transfusions when they experience severe anemia or are at risk for stroke. However, this option comes with a big catch: the risk of iron overload over time due to the accumulation of iron from transfused blood. This can lead to serious complications, like damage to the liver or heart.
Hydroxyurea is another significant tool in managing sickle cell disease. This medication works by increasing the production of fetal hemoglobin (HbF). Fetal hemoglobin mitigates sickling since it is less likely to polymerize and form rigid structures when oxygen levels dip. One of the benefits of hydroxyurea is its ability to reduce the frequency of pain crises, which are a hallmark of sickle cell disease. Nevertheless, like any treatment, it isn’t without its considerations. Patients may experience side effects, and there’s ongoing monitoring required to assess blood counts and any potential complications from long-term use.
Emerging Therapies and Research
The landscape of sickle cell treatment is continually evolving, with researchers tirelessly investigating new therapies. One promising direction involves gene therapy. This approach aims to correct the genetic defect itself, potentially restoring the patient's ability to produce normal hemoglobin. The idea is not just to treat the symptoms but to target the root cause of the disease.
There are also crisis prevention treatments being explored. One of these is L-glutamine, an amino acid that has shown promise in decreasing the frequency of pain episodes in some patients. It works by decreasing oxidative stress in the red blood cells, which in turn helps reduce sickling.
In addition, recent studies are investigating CRISPR technology as a means of genome editing. This cutting-edge research holds the potential for one-time treatments that could curtail or even cure sickle cell disease.
"Emerging therapies not only offer hope; they challenge the paradigms of traditional treatment, reorganizing the way we think about sickle cell management."
Furthermore, pain management strategies and educational programs about avoiding crises are also important elements of comprehensive treatment plans. Integrated care that pampers both the physical and mental aspects of living with sickle cell disease is vital for achieving better outcomes.
Overall, as research continues to forge ahead, the future looks hopeful for novel treatments that could significantly alter the trajectory of sickle cell disease, enhancing the quality of life for those who face this chronic condition.
Ongoing Research and Future Directions
Research into sickle cell disease is a constantly moving target. Scientists and medical professionals continue to examine how various mechanisms affect the severity of sickle-shaped red blood cells. This ongoing inquiry is vital, as it underpins the development of new treatment strategies and therapies that can profoundly improve patient outcomes.
One of the key areas of focus is the exploration of gene therapy approaches. Gene therapy has emerged as a promising area, providing a pathway to directly correct the mutations within the HBB gene responsible for producing hemoglobin S. Researchers are investigating methods of introducing healthy copies of the HBB gene into patients’ cells, aiming to restore proper hemoglobin function. This could, in theory, reduce or eliminate the production of sickled cells. The significance of such efforts cannot be overstated, as a successful gene therapy could completely alter the landscape of treatment for sickle cell disease, shifting from symptomatic management to potentially curative strategies.
Gene Therapy Approaches
In recent years, various advancements in gene therapy have shown promise for sickle cell disease. One notable method being studied is CRISPR-Cas9, a cutting-edge technology that allows precise modifications to DNA. Through this technology, researchers hope to correct the genetic mutation that causes hemoglobin S production directly in a patient’s own cells. This approach carries the potential not only to reduce the symptoms of the disease but could also lead to a long-term solution, possibly even a cure.
Another method under consideration is the use of lentiviral vectors. These vectors can deliver healthy genes into the patients’ hematopoietic (blood-forming) stem cells. Once treated, these cells would theoretically multiply and produce healthy red blood cells, helping to alleviate the effects caused by sickle-shaped cells. While this research is still in the experimental stages, preliminary results are showing encouraging outcomes, making it an area to watch. The benefits of such advances in gene therapy are enormous; if successful, they could drastically change the lives of those affected.
Understanding Disease Progression
Beyond gene therapy, there is a burgeoning interest in understanding disease progression. This line of research examines how and why the symptoms of sickle cell disease manifest. By grasping the intricacies of this progression, healthcare professionals may be able to predict complications more accurately and tailor treatments accordingly. One of the critical aspects researchers are focusing on is the microenvironment of the bone marrow and how it influences the development of sickle cell disease.
Research is also delving into the role of inflammation in the progression of sickle cell disease. It’s recognized that chronic inflammation is common in patients, but the full extent of its impact on overall health and disease severity is still under investigation. By meticulously studying these patterns, there can be a better understanding of how interventions might be designed to control inflammation, thereby potentially slowing disease progression.
In summary, ongoing research is crucial for deciphering the complex mechanisms that underlie sickle cell disease. Whether through novel gene therapies or understanding the paths of disease progression, each step forward has the potential to positively affect lives. Understanding these avenues not only enriches scientific knowledge but also carries with it the hope of better therapies for patients.
Finale
The conclusion of this article captures the essence of the exploration into sickle-shaped red blood cells, underscoring significant elements that warrant attention. Comprehending the complexities of sickle cell disease is not merely an academic exercise; it holds profound implications for patients and medical practitioners alike. Recognizing the genetic mutations responsible for the peculiar shape of these blood cells opens doors for enabling better treatment strategies and management options.
It’s critical to reiterate the multiple facets that influence the formation of these cells. From the genetic to the biochemical, and from environmental to clinical implications, each aspect interweaves tightly, creating a comprehensive picture of the condition. By synthesizing findings discussed throughout the article, we enhance our understanding of not only the mechanism but also the real-world impact on those who live with the disease daily.
"Understanding the causes of sickle cell disease can be likened to unraveling a knotted thread. Each twist and turn reveals important insights necessary for creating solutions to manage this condition effectively."
In essence, the significance is steeped in the potential benefits for a range of stakeholders: patients who seek effective control over their symptoms, researchers dedicated to pioneering novel therapies, and healthcare providers aiming for a more informed approach. Continued research into gene therapy and emerging strategies sheds light on the future direction of sickle cell management, driving home the point that every detail matters.
Recap of Key Points
- Sickle cell disease arises from mutations in the HBB gene, leading to the production of hemoglobin S.
- The formation of sickle-shaped red blood cells results from biochemical changes, particularly under low oxygen conditions.
- Environmental factors such as high altitude and temperature also play a role in exacerbating symptoms.
- Clinical implications include a range of complications that can significantly impact a patient’s quality of life.
- Emerging therapies and research offer hope for improved treatment options and disease management.
Final Thoughts on Sickle Cell Research
Continuous inquiry into the causes and implications of sickle cell disease cannot be overstated. As research evolves, the potential to unlock new understandings expands, promising advancements in treatment paradigms and preventative measures. A deeper knowledge of hemoglobin’s role and its mutation not only serves educational purposes but fosters empowerment—both for individuals living with the disease and the scientific community working tirelessly to mitigate its effects.