Where Are Stem Cells Found? An In-Depth Exploration
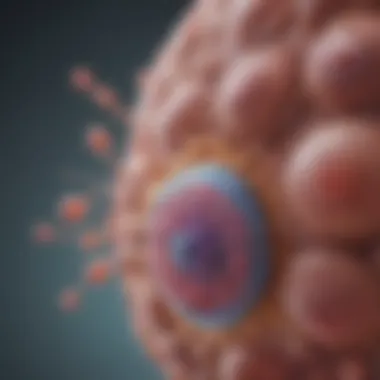
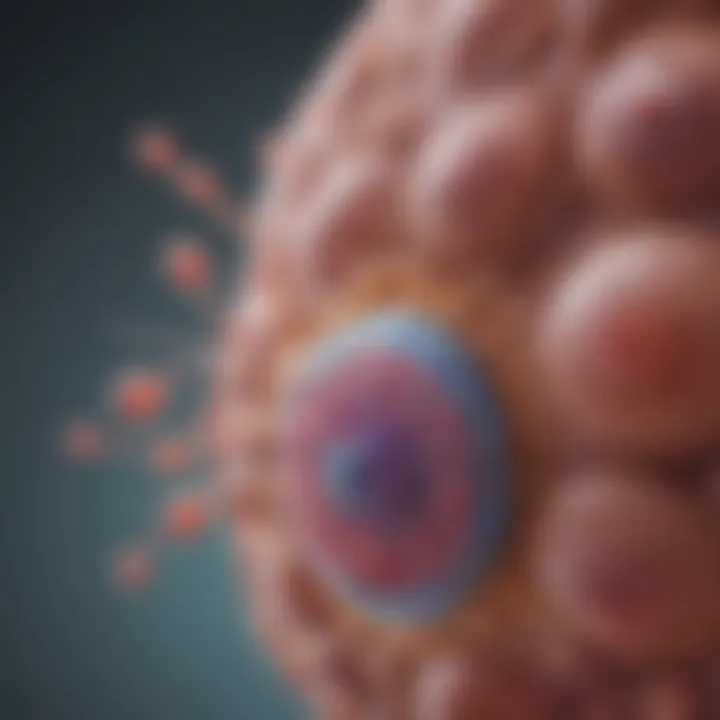
Intro
Stem cells have become a focal point of biological research due to their unique properties and potential for therapeutic applications. They exist in various locations within the human body and across different organisms. This exploration aims to unravel the complexities surrounding stem cells, including where they are found, types, origins, and their roles in health and disease.
This article is structured to provide insights into the biological significance of stem cells and their diverse locations, guiding readers through the existing literature and highlighting the ongoing research in the field.
Understanding where stem cells are located enhances our grasp of their biological roles, paving the way for advances in regenerative medicine and therapeutic interventions.
Research Methodology
In exploring stem cells, research has utilized a blend of observational techniques and experimental studies. The research design primarily centers on literature review and meta-analysis, focusing on peer-reviewed journals and credible sources like Nature and Cell. This approach offers a comprehensive view of where stem cells can be found and their implications in various medical contexts.
Description of Research Design and Approach
The methodology involves the following steps:
- Identification of Locations: Focusing on the major sites in human anatomy where stem cells are present, such as bone marrow, adipose tissue, and the brain.
- Types of Stem Cells: Distinguishing between embryonic stem cells, adult stem cells, and induced pluripotent stem cells.
- Applications: Reviewing studies that highlight the therapeutic potential of stem cells in treating diseases like cancer and neurodegenerative disorders.
Materials and Methods Used in the Study
The study also included an analysis of:
- Databases for locating stem cell research data, including PubMed and Scopus.
- Recent studies that examined stem cells' role in tissue regeneration.
- Case studies that illustrate the medical application of stem cells in clinical settings.
Discussion and Interpretation
The findings underscore the diverse locations of stem cells and their essential functions. For example, bone marrow houses hematopoietic stem cells that are crucial for blood cell formation, while the brain contains neural stem cells responsible for neurogenesis.
Interpretation of Results in Context of Existing Literature
The locations of stem cells have been extensively documented in the scientific literature. Recent studies have shown that the microenvironment of these cells plays a significant role in their functionality and therapeutic potential. A clear understanding of stem cell dynamics allows scientists to harness these cells effectively for regenerative medicine.
Implications for Future Research or Practical Applications
Looking forward, the exploration of novel stem cell sources, such as umbilical cord blood and dental pulp, offers promising avenues for research. Ongoing studies focus on optimizing stem cell therapies to treat various conditions, contributing to a deeper understanding of their capabilities and enhancing future applications in medicine.
Understanding Stem Cells
Understanding stem cells is crucial for grasping their role in biology and medicine. These cells are unique due to their ability to differentiate into various cell types and self-renew, making them vital in developmental processes, tissue repair, and regenerative medicine. This section will clarify what stem cells are, the different types, and their significant properties, showcasing their potential impact on health care and research.
Definition of Stem Cells
Stem cells are undifferentiated cells capable of giving rise to specialized cell types. They have two key characteristics: self-renewal, which allows them to divide and produce more stem cells, and differentiation, where they can develop into any cell type in the body. These cells exist in both embryos and adults, serving essential functions in growth, repair, and maintenance of tissues.
Types of Stem Cells
Stem cells can be categorized into three main types based on their origin and capabilities. Each type presents its unique profile, influencing their applications in research and therapy.
Embryonic Stem Cells
Embryonic stem cells (ESCs) are derived from the inner cell mass of blastocyst-stage embryos. They are pluripotent, meaning they can develop into virtually any cell type in the body. This feature is highly advantageous as it allows for extensive research and potential applications in regenerative medicine. The primary consideration surrounding ESCs revolves around ethical concerns related to embryo usage. Their potency offers tremendous possibilities, yet it raises questions about the future of stem cell practices.
Adult Stem Cells
Adult stem cells, also known as somatic or tissue-specific stem cells, are found in various tissues, including bone marrow, skin, and brain. Unlike embryonic stem cells, they have a more limited differentiation potential, typically giving rise to a specific cell type relevant to their location. Their accessibility and ethical standing make adult stem cells a popular area of research, particularly in regenerative therapies. However, their restricted potency can hinder broader applications in complex tissue regeneration.
Induced Pluripotent Stem Cells
Induced pluripotent stem cells (iPSCs) are adult cells reprogrammed to an embryonic-like state. This innovation allows them to gain pluripotency, similar to embryonic stem cells. iPSCs offer a remarkable advantage as they can be generated from a patient’s own cells, reducing the risk of immune rejection and obviating ethical conflicts linked to embryo use. However, the reprogramming process presents challenges regarding efficiency and the potential for tumorigenesis, which researchers are actively addressing.
Properties of Stem Cells
Stem cells share certain properties that distinguish them from other cell types. Two of these critical properties are self-renewal and potency, both essential for their biological functions.
Self-Renewal
Self-renewal is the ability of stem cells to undergo division and produce more stem cells. This characteristic is vital for maintaining the stem cell pool within an organism. High self-renewal capacity allows for sustained cell populations, which is critical during periods of growth or tissue regeneration. However, uncontrolled self-renewal can lead to tumorigenesis, making regulation an important area of study in stem cell research.
Potency
Potency refers to the capability of stem cells to differentiate into various specialized cell types. Stem cells vary in potency; totipotent stem cells can become any cell type in the organism, pluripotent cells can become nearly any cell type, and multipotent cells are limited to cell types within a particular lineage. Understanding potency helps guide the selection of stem cells for therapeutic approaches. The challenge lies in directing differentiation effectively to ensure desired outcomes in regenerative medicine.
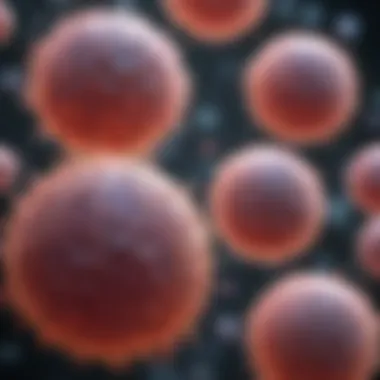
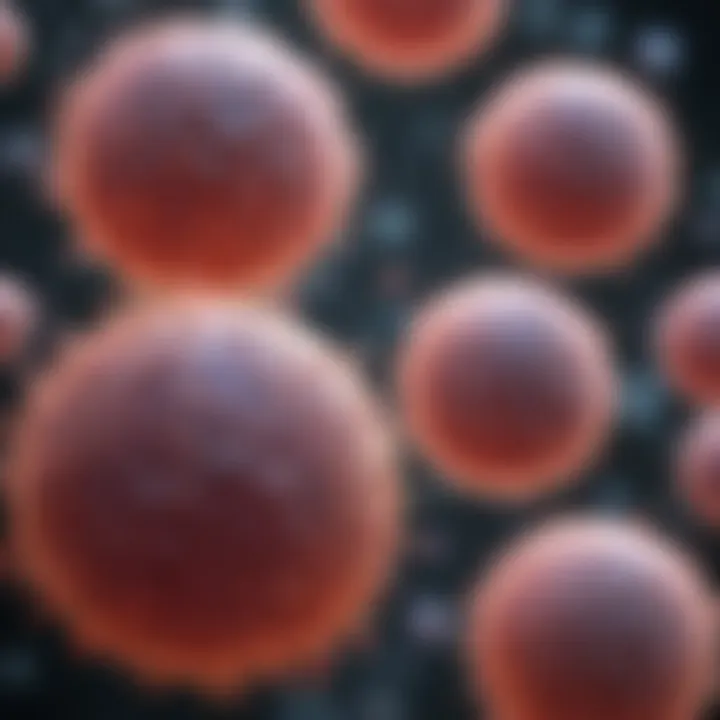
Stem Cells in Embryonic Development
Stem cells play a pivotal role in embryonic development. Their ability to differentiate into various cell types is fundamental for the formation of tissues and organs. Understanding the locations of these stem cells during early development provides insight into the mechanisms of growth and repair. This section will investigate the specific locations and functions of stem cells in the early embryo, focusing on the inner cell mass and blastocyst stage.
Location in Early Embryos
Inner Cell Mass
The inner cell mass (ICM) is a cluster of cells located within the blastocyst, an early stage of embryonic development. This group of cells is crucial as it contains pluripotent stem cells, which have the unique ability to develop into any cell type of the body. The ICM is significant because it serves as the precursor to the embryo itself. One key characteristic of the inner cell mass is its potential for differentiation.
While the ICM is a popular focus in stem cell research, it also raises ethical considerations. Those interested in the topic should note that while the ICM offers substantial research advantages, its extraction from embryos can present moral dilemmas, depending on one's philosophical or religious views.
Blastocyst Stage
The blastocyst stage occurs shortly after fertilization, approximately five to six days into development. At this stage, the embryo forms a hollow sphere encasing the inner cell mass. The blastocyst is important as it represents an evolutionary advantage that allows for implantation into the uterine wall, facilitating nutrient exchange. A notable characteristic of the blastocyst is its two key components: the trophoblast and the inner cell mass. The trophoblast will eventually form part of the placenta.
The blastocyst stage is also beneficial for research purposes. It is here where scientists can study early cell differentiation and the processes that lead to organ formation. However, the research surrounding this stage must be approached with caution. There are significant ethical considerations regarding the manipulation of blastocysts, as they are often derived from in vitro fertilization processes.
Role in Development
Stem cells in embryonic development are crucial for proper organ formation and structural organization. They determine the fate of cells through signaling pathways and interactions with surrounding tissues. Understanding their role not only enhances our knowledge of human growth but also paves the way for regenerative medicine applications. Stem cells' capacity to regenerate damaged tissues presents a promising avenue for treating various diseases and injuries.
"Stem cells are the body's raw materials — cells from which all other cells with specialized functions are generated. They divide very quickly and can transform into any type of cell." - (Source: en.wikipedia.org)
This focus on stem cells in embryonic development is vital for several reasons. It enables researchers to explore therapeutic strategies, such as delivering healthy stem cells to replace damaged ones, which could revolutionize treatments for degenerative diseases. The information from this segment underlines how integral stem cells are to early life, shaping the possible futures of individuals and their health.
Adult Stem Cells
Adult stem cells are a crucial area of study in the field of regenerative medicine and biology. These cells have the remarkable ability to regenerate and repair damaged tissues within the human body. Their importance lies in their role in maintaining homeostasis and tissue maintenance in adults. Understanding the locations and functions of adult stem cells can lead to significant advancements in medical therapies and treatments for various diseases.
Location in Various Tissues
Bone Marrow
Bone marrow is perhaps the most recognized source of adult stem cells. It is the primary site for hematopoiesis, the process through which new blood cells are produced. This location is significant because bone marrow contains hematopoietic stem cells, which give rise to red blood cells, white blood cells, and platelets.
The key characteristic of bone marrow is its rich vasculature, which is essential for the nourishment and growth of these stem cells. Bone marrow is a beneficial choice for sourcing adult stem cells due to its relative accessibility and the established techniques for harvesting these cells during clinical procedures. However, one unique feature is that extracting stem cells can result in pain or complications for the donor, which is a disadvantage that must always be considered in clinical practice.
Skin
Skin is another vital source of adult stem cells, particularly epidermal stem cells. These cells are located in the basal layer of the epidermis. The skin's role in protection and as the first line of defense against environmental stressors underscores the importance of skin-derived stem cells in regenerative medicine.
The key characteristic of skin is its constant state of regeneration, making it a popular choice for study. Skin has a unique feature as it can easily be biopsied with minimal discomfort, allowing for non-invasive procedures. A disadvantage, however, is that skin stem cells are limited in their potential compared to other sources, as they are often lineage-restricted to producing skin and hair follicles.
Muscle
Muscle tissue is also home to adult stem cells, known as satellite cells. These cells are involved in muscle growth and repair, particularly after injury. Satellite cells play a specific role in regenerating muscle fibers, making them indispensable for treatments related to muscle degeneration.
The key characteristic of muscle tissue is its ability to respond quickly to damage and initiate repair through activation of these satellite cells. This responsiveness makes muscle stem cells quite valuable in regenerative medicine. However, the unique feature lies in their limited number, as age can reduce the efficiency of muscle stem cells, presenting challenges for muscle recovery in older patients.
Brain
Lastly, the brain contains neural stem cells found in specific regions, especially the hippocampus. These adult stem cells contribute to neurogenesis, the process of generating new neurons. This is critical for learning and memory functions in adults.
The key characteristic of brain-derived stem cells is their ability to differentiate into various types of brain cells, including neurons and glial cells. This adaptability makes them an interesting focus in neurological research and potential therapies for brain injuries and neurodegenerative diseases. However, accessibility and difficulty in obtaining brain tissue for research purposes can be seen as significant challenges in studying these cells.
Significance of Adult Stem Cells
The significance of adult stem cells is underscored by their regenerative potential in various tissues. They are crucial in research and therapeutic contexts, particularly for conditions such as blood disorders, skin injuries, muscular dystrophies, and nervous system diseases.
Induced Pluripotent Stem Cells
Induced Pluripotent Stem Cells (iPSCs) are a significant area of study within stem cell research. They are adult cells that have been genetically reprogrammed to an embryonic stem cell-like state. This technology allows for the potential use of patient-specific cells for therapeutic needs without the ethical concerns associated with embryonic stem cells.
The importance of iPSCs lies in their ability to differentiate into any cell type in the body, providing exciting possibilities for regenerative medicine. For example, iPSCs could be used to generate tissues for grafting, develop drugs, and create models for studying diseases.
"Induced pluripotent stem cells represent a remarkable advancement in regenerative medicine and personalized therapy."
Creation and Location
The process of creating iPSCs involves several steps. Researchers typically begin by taking somatic cells from a patient, which could be skin cells or blood cells. Then, they introduce specific genes, often using viral vectors, that are crucial for maintaining the pluripotent state. The Oct4, Sox2, Klf4, and c-Myc genes play a key role in reprogramming these cells to become iPSCs.
Once generated, iPSCs are usually cultured in a laboratory setting to maintain their pluripotent characteristics. They can essentially exist in a dish until needed for either research or potential therapeutic applications. The reprogrammed cells can be localized and sustained in different forms of culture systems, which are carefully monitored to ensure optimal conditions for stem cell growth.
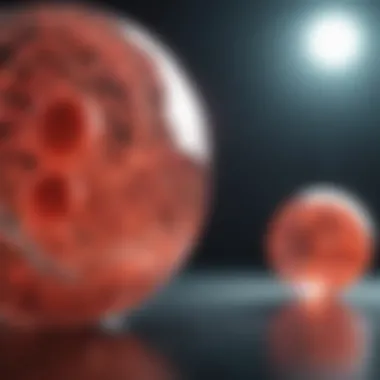
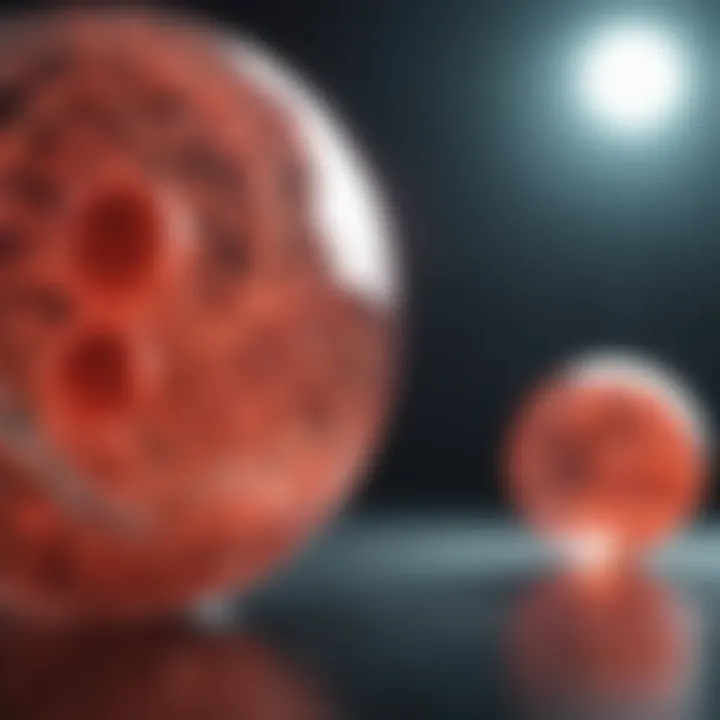
Applications of Induced Pluripotent Stem Cells
The applications of iPSCs are vast and are still being explored. Here are some of their key uses:
- Regenerative Medicine: iPSCs can generate various cell types for treating degenerative diseases, such as Parkinson's or spinal cord injuries. It allows for the repair of damaged tissues.
- Drug Development and Testing: By creating patient-specific iPSCs, researchers can test drugs more effectively, determining responses based on individual genetic backgrounds.
- Disease Modeling: iPSCs allow scientists to create cell models of specific diseases, providing insight into disease mechanisms and potential therapeutic targets.
- Tissue Engineering: iPSCs may aid in developing tissues that can be transplanted into patients, reducing the risk of rejection since they are derived from the same individual.
Stem Cell Niches
Stem cell niches are specialized microenvironments that provide the necessary support for stem cells. They are crucial for maintaining the balance between stem cell proliferation and differentiation. The concept of a niche underscores how stem cells are not simply present in isolation. Instead, their location within these niches helps define their fate and function.
Definition and Importance
Stem cell niches are specific anatomical areas where stem cells reside. These niches provide a regulatory context that promotes self-renewal and differentiation when needed. They are composed of a variety of cell types, extracellular matrix components, and signaling molecules. The importance of stem cell niches in this context cannot be understated. They ensure that stem cells remain undifferentiated when necessary while also providing the signals that trigger differentiation when the organism requires it. This dual function is essential for development, healing, and homeostasis.
Examples of Stem Cell Niches
Hematopoietic Stem Cell Niche
The hematopoietic stem cell niche is located primarily within the bone marrow. This niche plays a vital role in the production of blood cells. The key characteristic of this niche is its complex architecture, which includes different cell types such as osteoblasts and endothelial cells. This complexity supports the maintenance and function of hematopoietic stem cells. One of the unique features of the hematopoietic niche is the presence of signaling molecules like cytokines that regulate the balance between stem cell maintenance and differentiation. The advantages of this niche include its accessibility for clinical applications, such as bone marrow transplants. However, its complexity can also pose challenges in understanding how to manipulate it for therapeutic purposes.
Neural Stem Cell Niche
Neural stem cell niches are found in specific regions of the central nervous system, notably the subventricular zone and the dentate gyrus of the hippocampus. These niches contribute to neurogenesis, which is the process of generating new neurons. A key characteristic of the neural stem cell niche is its ability to respond to various stimuli, including injury, which can trigger neurogenesis. This adaptability makes the neural niche an interesting area for research, particularly in aging and neurodegeneration. A unique feature is the interaction between neural stem cells and their environment, which involves signaling pathways that are critical for proper function. One challenge is that the neurogenic capacity of these niches decreases with age, which has implications for potential therapies.
Mesenchymal Stem Cell Niche
Mesenchymal stem cells are predominantly found in the bone marrow, adipose tissue, and even muscle. The mesenchymal stem cell niche is significant for tissue repair and regeneration. A notable characteristic of this niche is its diverse cellular environment, which supports the differentiation into multiple cell types, including bone, cartilage, and fat cells. The unique feature of the mesenchymal niche is its ability to interact with various growth factors and cytokines which can influence the fate of the stem cells. This flexibility is beneficial for regenerative medicine. However, one disadvantage is the difficulty in isolating and culturing mesenchymal stem cells from their niches due to the heterogeneous environments they exist in.
"Understanding stem cell niches is fundamental to harnessing their potential in medicine and biology."
In summary, stem cell niches are essential for the functioning of stem cells across different contexts. Each niche demonstrates distinct characteristics and advantages, yet also presents challenges that need to be addressed for future applications in research and therapy.
Locations of Stem Cells Across Organisms
The exploration of stem cells across various organisms emphasizes their fundamental role in growth, development, and regeneration. This section outlines where stem cells can be found in different biological categories, including plants, invertebrates, and vertebrates. Understanding these locations highlights their significance, both in evolutionary contexts and their potential applications in biotechnology and medicine. The diversity of stem cell locations underscores the adaptability of life forms and the complexity of biological systems.
Stem Cells in Plants
In plants, stem cells are primarily located in specific regions known as meristems. These areas are crucial for growth and development. The two major types of meristems are apical meristems and lateral meristems.
- Apical Meristems: Found at the tips of roots and shoots, these stem cells contribute to the elongation of the plant and the formation of new leaves, flowers, and stems.
- Lateral Meristems: Responsible for secondary growth, these cells contribute to the thickening of stems and roots.
Plant stem cells can differentiate into various cell types, enabling plants to adapt to changing environments. For instance, when a plant is injured, stem cells may activate to produce new tissues for repair. This regenerative capability is key for understanding how plants survive adverse conditions.
Stem Cells in Invertebrates
Invertebrates, such as hydra and planarians, possess remarkable regenerative abilities. Their stem cells can be found in various tissues and are often referred to as neoblasts. In planarians, neoblasts are distributed throughout the animal's body and serve as a source for regeneration. When a planarian is cut, these stem cells migrate to the wound site and differentiate into various needed cell types, including muscle, nerve, and skin cells.
In addition, hydra's stem cells, located in the interstitial tissue, exhibit notable plasticity. They can give rise to epithelial cells, neurons, and even gametes. This indicates a high level of versatility and a robust capacity for regeneration, which offers insights into cellular processes that may have implications for human medicine.
Stem Cells in Vertebrates
In vertebrates, stem cells are found in various locations, each serving distinct purposes within the organism. Some key areas include:
- Bone Marrow: Contains hematopoietic stem cells responsible for producing blood cells.
- Skin: Contains epidermal stem cells that regenerate skin and hair.
- Muscle: Skeletal muscle houses satellite cells that repair and regenerate muscle tissues.
- Brain: Neural stem cells located in areas like the hippocampus contribute to neurogenesis, the process of creating new neurons.
These locations highlight not only the diversity of stem cell types but also their specific roles in maintaining homeostasis and facilitating repair mechanisms. The understanding of stem cell locations is vital for advancing therapeutic approaches for various conditions, including degenerative diseases and injuries.
In summary, locations of stem cells across organisms provide valuable insights into regeneration, adaptability, and potential applications in regenerative medicine.
Clinical Applications of Stem Cells
Stem cell research has vast implications for medicine. The exploration of clinical applications illuminates how stem cells can be utilized in therapies and treatments. Understanding these applications is vital as they exemplify not just the potential of regenerative medicine but also address critical needs in health care. In this section, we will delve into current therapies available today, including bone marrow transplants and stem cell therapy for degenerative diseases, and explore future directions in stem cell research that promise even more innovations.
Current Therapies
Bone Marrow Transplants
Bone marrow transplants are among the oldest and most established uses of stem cells in treating diseases, particularly certain cancers such as leukemia. This therapy involves replacing diseased or damaged bone marrow with healthy cells. The key characteristic of bone marrow transplants is their ability to restore blood cell production. Thus, they are a valuable option for patients whose bone marrow does not produce enough healthy blood cells.
A unique feature of this therapy is the source of stem cells, which can come from the patient (autologous) or a donor (allogeneic). This flexibility expands patient access to treatment. However, this procedure is not without risks. Complications may arise, including infections and graft-versus-host disease, where the transplanted immune cells attack the recipient’s body. Despite these challenges, the potential for recovery and improvement in quality of life makes bone marrow transplants a well-established therapeutic approach for various life-threatening conditions.
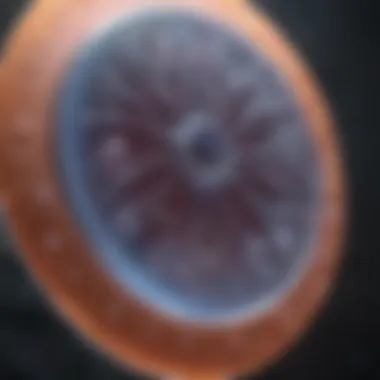
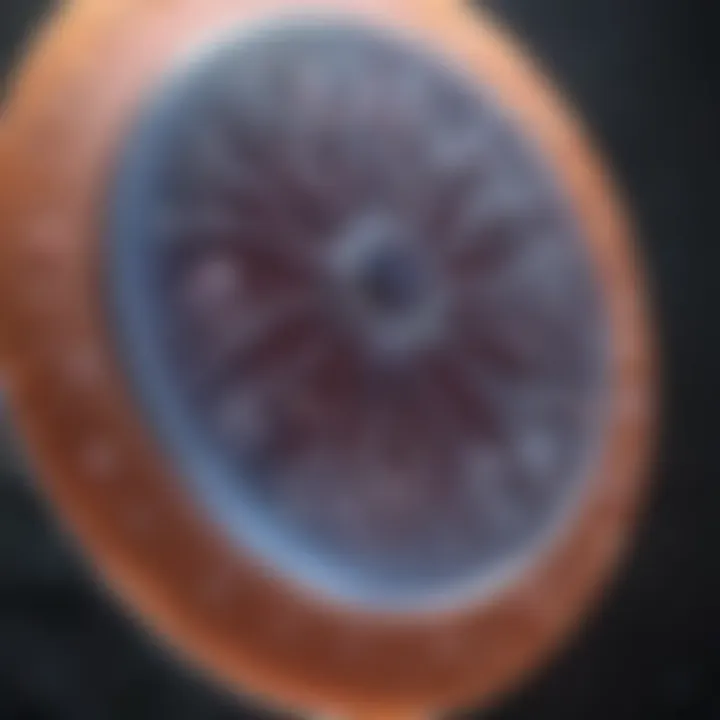
Stem Cell Therapy for Degenerative Diseases
Stem cell therapy for degenerative diseases represents a significant advancement in medical science. This therapy aims to repair or replace damaged cells in conditions such as Parkinson’s disease, multiple sclerosis, and other chronic illnesses. The key feature of this type of therapy is its focus on regenerating tissues and restoring function, which can lead to substantial improvements in patient well-being.
One unique aspect of stem cell therapy for degenerative diseases is the use of induced pluripotent stem cells (iPSCs), which can be created from a patient's own cells. This means fewer issues with rejection since the cells are genetically identical to the patient’s. However, the long-term effects and efficacy of these therapies are still under investigation. The challenge remains the need for rigorous clinical trials to establish their safety and effectiveness. This makes the continued exploration of stem cell therapies not only crucial but also an exciting frontier in medical research.
Research Directions
The future of stem cell research is poised for significant developments. Emerging technologies are likely to enhance our understanding and application of stem cells in various therapies. For instance, advancements in gene editing technologies, like CRISPR, offer the potential to correct genetic defects at the stem cell level. Moreover, research into combining stem cell therapy with other treatments, such as immunotherapy, may yield groundbreaking results.
Additionally, new applications in tissue engineering are being explored. Researchers aim to develop methods to grow complex tissues and organs for transplantation, which could mitigate organ shortage issues. Furthermore, ongoing studies into the ethical implications surrounding stem cell research and therapies need careful consideration as public interest and funding evolve.
"As we advance in the realm of stem cell research, our pursuit for ethical, effective, and accessible treatments continues to shape the future of medicine."
Overall, clinical applications of stem cells highlight their crucial role in regenerative medicine. Continued exploration promises advancements that could transform treatment protocols and improve outcomes for patients worldwide.
Ethical Considerations in Stem Cell Research
The examination of ethical considerations in stem cell research is crucial in understanding the implications of scientific advancements in this field. Stem cell research promises significant medical advancements such as treatments for degenerative diseases and potential cures for conditions that affect the quality of life. However, these advancements come with complex ethical concerns, particularly regarding embryonic stem cells, which have become a focal point of debate.
Debates Surrounding Embryonic Stem Cells
Embryonic stem cells are derived from early-stage embryos, a process that raises profound ethical questions. Some argue that using embryos for research is morally unacceptable, as it involves the destruction of potential human life. The perspective often stems from various cultural, religious, and personal beliefs regarding when life begins. Critics of embryonic stem cell research believe that it commodifies human life, treating embryos as mere resources for scientific pursuit.
On the flip side, advocates argue that the potential therapeutic benefits of embryonic stem cells outweigh the moral concerns regarding embryos. They posit that these stem cells are essential in researching and developing treatments for conditions like Parkinson’s disease, diabetes, and spinal cord injuries. The ongoing debate centers around finding a balance between scientific progress and ethical integrity, as both sides present compelling arguments regarding the value of life and medical innovation.
Regulatory Framework
The regulatory framework surrounding stem cell research is vital in shaping ethical practices in the field. Different countries have different regulations concerning embryonic stem cell research, reflecting the diverse societal values. For instance, in the United States, the National Institutes of Health (NIH) provides guidelines, but state laws can vary significantly, leading to a patchwork of regulations. Countries like the United Kingdom have more comprehensive regulations in place, ensuring that research is conducted ethically and responsibly.
These regulations often require researchers to obtain informed consent from donors, ensuring that participants are fully aware of the implications of their contributions. Furthermore, funding for stem cell research can be influenced by ethical guidelines, restricting public funding for research involving human embryos in some regions.
As research evolves and new technologies emerge, the regulatory framework must adapt accordingly. Keeping pace with scientific advancements allows for responsible exploration while minimizing ethical conflicts. This dynamic nature of regulation aims to address both the necessity of progress in stem cell research and the critical need for ethical oversight throughout the process.
"The ethical landscape of stem cell research is continually evolving, as both scientific knowledge and societal values shift over time."
In summary, the ethical considerations surrounding stem cell research are multifaceted. The debates on embryonic stem cells highlight the conflict between scientific progress and moral standards, while the regulatory framework seeks to establish a responsible path forward. As we continue to explore this rich field, ongoing dialogue among scientists, ethicists, and the public will be essential to navigate these complex issues.
Future Directions in Stem Cell Research
The exploration of future directions in stem cell research holds immense significance as it guides scientists and medical professionals toward innovative therapies and solutions for a range of diseases and conditions. The field is evolving rapidly, and understanding where it is headed can inform researchers, students, and healthcare practitioners on potential breakthroughs that may transform medical practices and patient care.
Emerging Technologies
Emerging technologies in stem cell research are poised to revolutionize the field. One key area of focus is the advancement of CRISPR-Cas9 gene editing. This technology enables precise modifications of genetic material, which opens avenues for correcting genetic disorders at the stem cell level.
- Organ-on-a-chip systems are another groundbreaking innovation. These devices mimic human organ systems, allowing researchers to study disease processes and test the efficacy of stem cell therapies more effectively.
- 3D bioprinting is another exciting development. Scientists are utilizing this technology to create complex tissue structures from stem cells, enabling regenerative medicine to progress toward functional organ creation.
- Single-cell sequencing enhances our understanding of stem cell heterogeneity. It allows researchers to analyze individual stem cells, leading to insights that can improve therapeutic targeting and efficacy.
With each advancement, the potential for more effective treatments and a deeper understanding of stem cell biology grows.
Potential New Applications
The potential applications of stem cells are vast and multifaceted. These applications focus not only on repairing damaged tissues but also on preventing diseases before they develop.
- Regenerative medicine stands out as a critical area. Stem cells could regenerate damaged heart tissue after a heart attack or repair spinal cords after injury.
- Cell therapy is being closely studied for its role in treating autoimmune diseases. For instance, utilizing mesenchymal stem cells to modulate immune responses may provide new avenues in managing conditions like rheumatoid arthritis.
- Cancer treatment is another promising domain. Researchers are investigating ways to harness stem cells to deliver targeted therapies directly to tumors, potentially reducing damage to healthy tissue and increasing the effectiveness of treatments.
- Personalized medicine is also on the horizon. Stem cells derived from a patient’s own tissues can be engineered to create tailored treatments that minimize adverse reactions and maximize therapeutic outcomes.
"Stem cell research promises to significantly alter the landscape of medicine, presenting opportunities to treat and possibly cure previously unmanageable diseases."
Continued investment and collaboration in this area could yield breakthroughs that reshape healthcare globally.
Epilogue
Recap of Key Points
The article encapsulates several important findings:
- Diverse Locations: Stem cells reside in diverse tissues, ensuring a supply for repair and regeneration. Key areas include bone marrow, brain, skin, and muscles.
- Types of Stem Cells: Different stem cells such as embryonic, adult, and induced pluripotent cells have unique characteristics, origins, and functions.
- Clinical Relevance: Stem cells are increasingly recognized for their therapeutic potential, particularly in treating degenerative diseases and in regenerative medicine.
- Ethical and Future Considerations: Ethical debates continue surrounding the use of embryonic stem cells, while emerging technologies promise new avenues for research and therapy.
The Importance of Continued Research
Continued research in the field of stem cells is vital for several reasons. First, as science progresses, our understanding of stem cells deepens, providing insights that can enhance clinical applications. By identifying how stem cells function in their niches and how we can manipulate them effectively, researchers can unlock new therapies for conditions that are currently untreatable.
Second, ongoing studies help to address the ethical concerns surrounding stem cell research. Open dialogue and findings can lead to more informed discussions and policies that guide responsible research.
Lastly, advancing technologies in stem cell research can pave the way for revolutionary treatments. Emerging techniques, such as gene editing and bioengineering, hold promise for enhancing the functionality of stem cells, resulting in greater innovation in medical treatments.
"The exploration of stem cells continues to be one of the most dynamic fields in biological research, with the potential to redefine medical care and rejuvenation therapy."
In summary, a thorough understanding and continued exploration of stem cells remains not only significant but also essential as we navigate the complexities of biology, medicine, and ethics.